I am of anthropic origin, very little is said about me, and everyone is convinced that they understand me perfectly. What am I? Climate change, of course!
All the energy transition scenarios for 2050 in France (ADEME, RTE, negaWatt) assume a relatively large – if not exclusive – share of renewable energy in the French energy mix. As a reminder, the current French energy mix is made up of almost 45% fossil fuels (when the global mix is over 80%). Photovoltaic energy, generated from photovoltaic cells installed on photovoltaic panels, is one of the renewable energies. Agriculture is one of the sectors that has long been interested in the production and use of these renewable energies, directly on the farm. Between small-scale wind power, photovoltaics, solar thermal energy, methanisation and wood energy, you will admit that there is plenty to do.
This blog post focuses on just one of these energies – photovoltaic energy – produced in the same environment as agricultural production. We will refer to this as agrivoltaism. This blog post is not a complete state of the art of what agrivoltaism is. Others – ADEME in particular – have done so before me. The idea here is to question the uses and issues of this new agricultural practice and to try to understand whether it should be seen as a new form of diversification for the agricultural landscape or whether, on the contrary, the threat it poses to the agricultural sector should push us to turn away from it. The answer is obviously not binary…
Agrivoltaism was even mentioned by Emmanuel Macron during the presidential debate between the two rounds, without really knowing how it came about. In short, it was time to take a look at it.
As usual, for the readers of the blog, this article is the result of video interviews with players in the sector (whose names you will find at the end of the article) whom I thank for the time they were able to give me. Several articles, reports and seminars have allowed me to complete the feedback from the interviews.
Some photovoltaic developers and other actors in the sector did not wish to discuss with me…
Enjoy your reading!
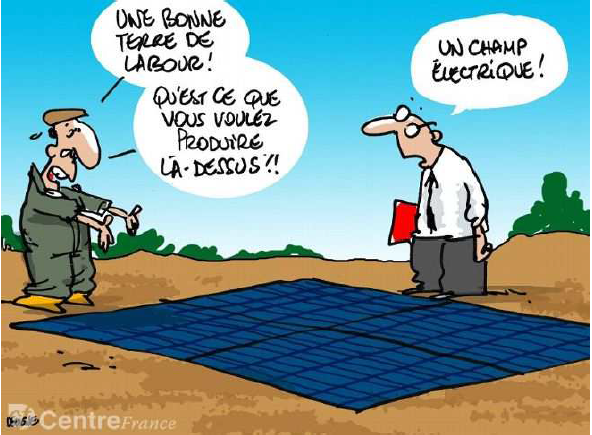
Translation :
- “Good soil for ploughing! What do you want to produce on it?”
- “An electric field”
From Photovoltaics to Agrivoltaics
A quick update on photovoltaics
Let’s go over some vocabulary to make sure we are talking about the same thing (Figure 1)! A photovoltaic system (or photovoltaic tables – I will use this term in the file) is composed of a set of photovoltaic panels. Each panel is in fact a photovoltaic module put back together. And each photovoltaic module is made up of a set of photovoltaic cells that produce electrical energy. By abuse of language – especially in the agricultural field – you may hear people talk about photovoltaic panel installations when in reality, it is actually assemblies of panels (which are therefore called photovoltaic tables) that are installed. I will therefore use the terms photovoltaic tables and photovoltaic panels in this dossier to mean more or less the same thing.
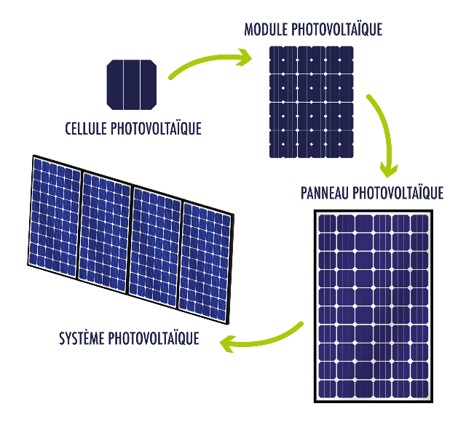
Figure 1: From the photovoltaic cell to the photovoltaic panel
Be careful to use the term “photovoltaic panel” (or “solar photovoltaic panel”) and not just “solar panel”. Solar panels do exist but, unlike photovoltaic panels, they are not used to produce electricity from solar energy but heat (again from solar energy). Solar panels are mainly used for domestic heating (e.g. heating your hot water tank).
It is therefore the photovoltaic cell that converts solar energy into electrical energy. The photovoltaic cell is usually composed of two silicon-based semiconductor layers – the N-doped silicon layer (N-layer) and the P-doped silicon layer (P-layer) as shown in Figure 2. The N-layer is electron surplus (i.e. negatively charged) and the P-layer is electron deficient (i.e. positively charged). The NP transition layer (or junction layer) shown in the figure is not a slab of material (as are the two silicon layers) but rather an electric field resulting from the bringing together of the two differently charged silicon layers (a bit like a battery). For information on the junction layer or NP transition, we speak of a homojunction if the two silicon layers are crystalline silicon, and a heterojunction if one of the two layers is crystalline silicon and the other amorphous silicon.
When photons of light pass through the N-doped silicon layer, the energy of the photons is transferred to the electrons, which can then break free from the attraction of the silicon core around which they orbit. The electrons in the N-layer thus move to the P-layer and are collected at a conductive plate which then returns them to the N-layer. It is the movement of the electrons back to the N layer that creates electricity. The anti-reflection layer on top of the photovoltaic cell prevents too many photons from being reflected off the surface of the cell and passing through the cell.
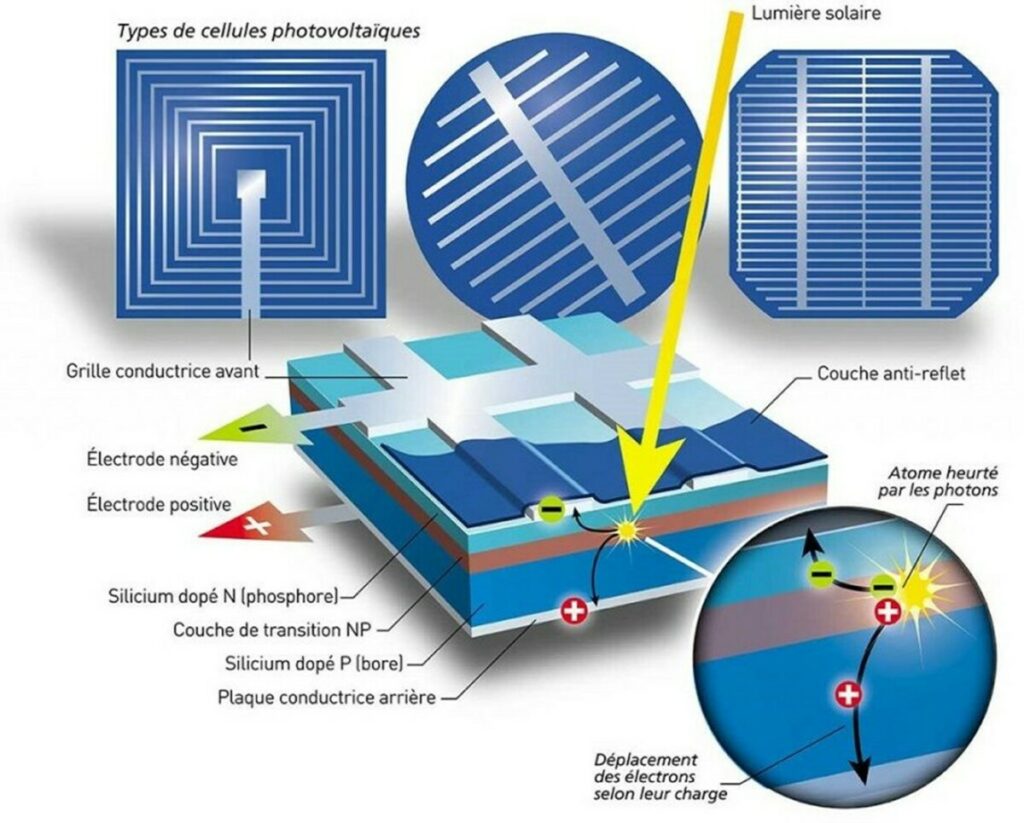
Figure 2. Details of a photovoltaic cell
We often see quite contradictory information on photovoltaic panels, so sensitive are the issues of energy mix for some. I’ll come back here quickly to some additional details to keep in mind:
- Photovoltaic panels lose about 2% of their power when they are installed and then 0.5% of their power every year. Photovoltaic panels are generally guaranteed for 20 years (some panels are guaranteed for longer than that). It is important to understand that a certain efficiency of the panels – usually at least 80% – is guaranteed for these 20 years. The panel can continue to function after that time, just with a lower efficiency.
- Photovoltaic panels do not contain rare earths (there are only rare earths in the batteries and the panels can be connected to local batteries, especially for self-consumption). Silicon is abundant on earth. Silicon is derived from the silica found in sand and quartz (which must then be refined and purified).
- The current energy yield of photovoltaic cells is around 20%, i.e. the cells are able to convert 20% of the solar energy they receive into electrical energy. This efficiency is steadily increasing. Some conventional ground-mounted solar parks are dismantled and reassembled with new, more efficient panels.
- We speak of 1st generation photovoltaic cells (based on crystalline silicon), 2nd generation photovoltaic cells (based on amorphous silicon in thin layers) and 3rd generation photovoltaic cells (based on organic compounds – we will come back to this a little later).
A few notations and orders of magnitude to conclude:
- The term “peak”, e.g. MegaWatt peak (MWp) or GigaWatt peak (GWp) is basically the maximum power that a photovoltaic installation can produce.
- A megawatt hour (MWh) is the amount of energy produced in one hour by one megawatt (MW).
- A conventional ground-mounted photovoltaic plant on a surface of 1 hectare can produce roughly 1MWp
- 1 TeraWatt = 1000 GigaWatt = 1.000.000 MegaWatt
The objectives of the Multiannual Energy Programmes (MPE)
The Multiannual Energy Programmes (MPE) are tools for steering French energy policy. The MPEs were created in 2015 following the law on the energy transition for green growth (LTECV). Bear in mind that the government is currently preparing its future energy and climate programming law (LPEC) which will replace the previous LTECV. Future multi-annual energy programming (notably the MPE 3, which will run from 2024 to 2033) will have to be compatible with the LPEC once the latter is finalised.
The MPEs contain a number of instructional strands, one of which is of particular interest to us here and relates to the development of renewable energy exploitation. At the end of the third quarter of 2021, the photovoltaic installations present in France were capable of generating 12.3 GW, i.e. about 3% of French electricity consumption (I am talking about electricity consumption and not energy consumption – not all energies are electric). This means that about 50% of the 2023 target has been reached so far (around 24GW is required for photovoltaics (Figure 3). The targets for the development of photovoltaic electricity production to be reached by 2028 are between 35.1 GW for the low option and 44 GW for the high option (the figure below shows the figure of 41 GW). So there is still some way to go…
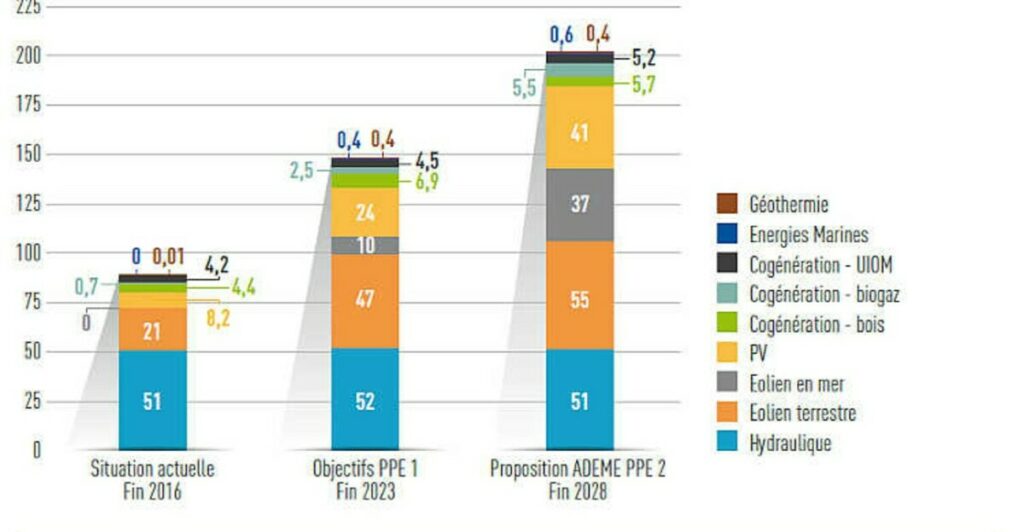
Figure 3: Electricity generation targets (in GW) for the Multiannual Energy Plans (MPE)
Plant and photo period
Photosynthesis is the absolutely fantastic reaction that allows plants to convert the sun’s energy into chemical energy for their functioning. It is within their leaves, in the membrane of the chloroplasts, that we find the chlorophyll pigments that carry out this photosynthesis. Over the entire electromagnetic spectrum of light, the main chlorophyll pigments a and b absorb wavelengths in the red and blue dominants of the visible spectrum in a photochemical reaction so that the plant can use the solar energy received (chlorophyll absorbs very little of the green wavelengths, which is why we see green leaves in spring and summer).
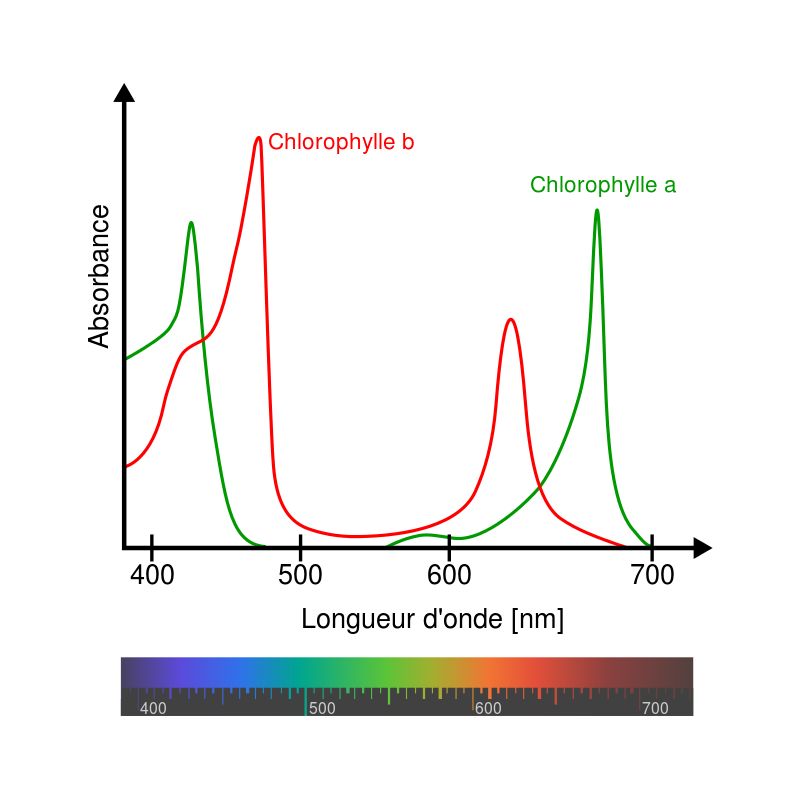
Figure: Absorbance spectrum of chlorophyll
Photosynthesis in plants takes place when the light compensation point is reached. This means that there is enough light energy for photosynthetic activity to produce more oxygen than the plant needs for its respiration. A widely used argument for agrivoltaism, there is also a saturation point beyond which the additional light input is no longer used by the plant anyway (the photosynthesis curve stabilises), and can thus be reused to produce electrical energy. It should also be noted that too high intensities (whether in terms of light quantity, external temperature, or quantity of surrounding CO2) can render certain reactions limiting and reduce the speed of photosynthesis in certain plant organisms – there would be a kind of saturation of photosynthesis in these stressful environments for the plant (photo-inhibition would refer to an excess of light). We could also add that as chlorophyll pigments do not use the entire light spectrum, it would theoretically be possible to design devices that would leave the ‘good’ wavelengths to the plants and that would use the unused wavelengths to produce electrical energy (more on this later).
What is agrivoltaism ?
Agrivoltaism can be defined quite simply as an agricultural practice that seeks to ensure both agricultural production (plant or animal – in the latter case we will speak of livestock agrivoltaism) and electricity production from photovoltaic panels, on the same agricultural surface. The term “agrivoltaism” is preferred in France (first used by Christian Dupraz – researcher at INRAE – in 2011), but similar dynamics can be found under other terms such as agro-photovoltaic, agrivoltaic systems, or photovoltaic agriculture. Numerous public and private actors have proposed definitions, in particular structures such as France Agri Voltaisme, the National Assembly (following its Flash mission), or more recently ADEME. The AFNOR has also proposed a framework for labelling agri-voltaism projects (work on agri-voltaism for livestock is underway). Good luck, however, in finding a precise, concrete and objective definition. The tones given to these definitions are generally rather honeyed and terribly general. You will hear about “significant production”, “acceptable degradation of production”, “synergy”, “cohabitation” or “co-activity”. The desire is clearly not to offend the players around the table.
The question that arises in reality is to know whether such and such a photovoltaic project should be considered as agrivoltaism or rather as a disguised or alibi project. And the stakes are high, as we will discuss in the rest of this report. Some actors allow themselves to compare agrivoltaism to agro-forestry in the sense that agrivoltaism could be seen as a two-storey crop, where the upper vegetation is replaced by mineral (steel galvas with photovoltaic tables). Everyone will judge the relevance or not of this comparison…
A photovoltaic project on agricultural land (whether it is considered as agrivoltaism or not) includes at least :
- Agricultural crops under panels (in crop production) and usually grassland (in animal production)
- Photovoltaic tables, each containing photovoltaic cells capable of producing electricity
- A more or less complex infrastructure to hold the photovoltaic tables (steel bars, piles, ground anchors, etc.)
- An inverter to transform the direct electric current generated by the photovoltaic cells into alternating current so that this current can be integrated into the grid and be reused by other people. Keep in mind that the inverter can work without a power grid and power for example a battery on site. As a rule, photovoltaic tables are connected to the electricity grid because the electricity is sold back to the grid (and since the voltage on the grid must be low, alternating current is preferred, despite Thomas Edison’s words).
- A set of technical and logistical infrastructures (fence to protect the photovoltaic plant, access roads….)
Should we really oppose agricultural production and electricity production? Should we make land sacred for agriculture and, depending on political decisions, land-use planning and our ambitions, use other land to produce electricity with more or less conflict? Or should the two coexist? As usual, the answers are not binary and I will try my best to give you the keys to reading the whole dossier.
I will only focus here on the installation of photovoltaic projects linked to a certain agricultural production. I will therefore ignore the installation of photovoltaic panels on the roofs of buildings or sheds, which can roughly be considered as classic photovoltaics.
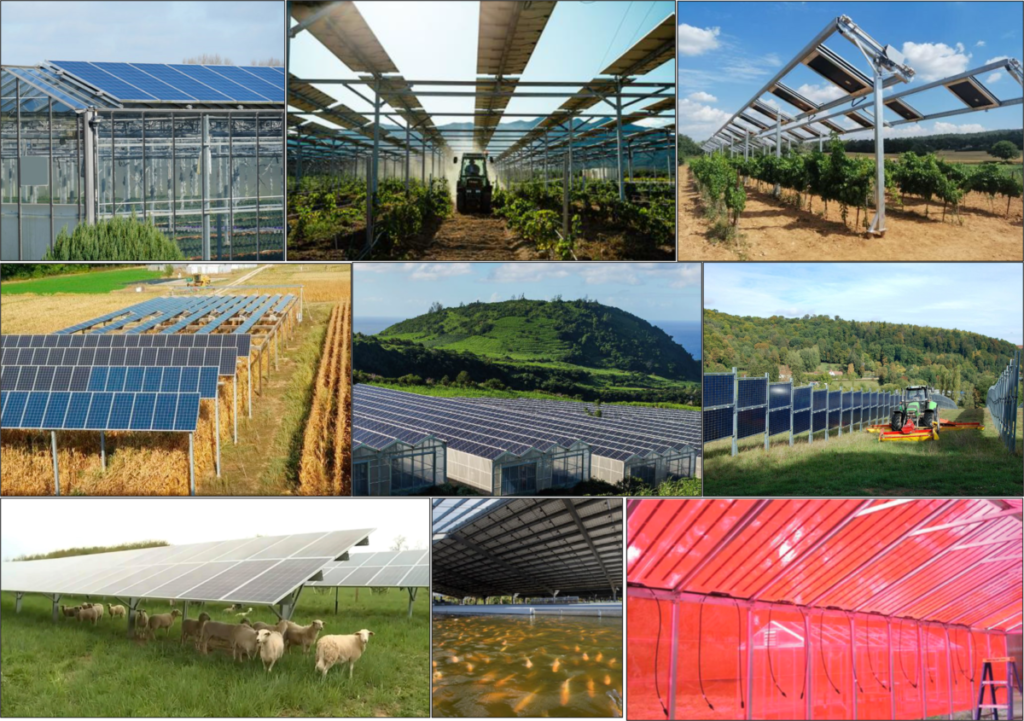
Figure 4. From left to right and top to bottom: photovoltaic greenhouse, dynamically controlled shades, sliding shades, fixed shades, multi-hutches, bifacial panels, sheep under fixed panels, fish under fixed panels, magenta greenhouse.
Issues and services of agrivoltaic projects
A change of agronomic reference point
The objective of this section is not to give a very exhaustive overview of all the services provided by photovoltaic installations; firstly because such a complete knowledge does not exist in the literature, but also because some technical reports will have already tried to centralise the existing knowledge. Instead, let’s try to come back to some general conclusions
The first thing to say is that current experiments seem to show a neutral or negative effect of photovoltaic installations in agriculture (ADEME, 2021). The complexity lies in the fact that the results can be very heterogeneous and are difficult to compare between the studies carried out (a project in apples will not have the same meaning in Cavaillon or in Normandy), and that the work is rather unevenly distributed over the territory, which limits even more the extrapolation of results and the comparison of different agro-pedo-climatic conditions
Crop production
It is difficult to conclude from the studies whether a particular crop is compatible with an agrivoltaic system. In terms of crops covered by photovoltaic tables, there are many experiences (Figure 4). However, there is mainly work on high value-added perennial crops (vines and orchards [apple, apricot, cherry, etc.] and annual crops grown in greenhouses as part of market gardening activities (tomatoes, cucumbers, etc.). Field crops are less targeted for the time being, already because the surfaces are larger, but perhaps also because cereal crops are harvested in late spring or early summer – periods during which the main climatic risks have not yet occurred. Field crops are also agricultural areas subject to more or less long crop rotations, and their management under panels could be all the more complex. Let’s also add the mechanisation constraints which do not help the matter (even if some players propose technologies in this sense).
From shade to light and water management
Shade and light are two sides of the same coin that photovoltaic tables are committed to sharing between agricultural production and electricity production. Shading is interesting for plants insofar as it allows the temperature of the canopy to be lowered (and thus reduces the evapotranspiration phenomena of the plants) and, when the light is too high, to limit the periods of photo-inhibition that we mentioned above in the introduction. Rather than states of light and shade, it is sometimes more in terms of alternation that we need to think. With the sun’s path, the photovoltaic tables create periods of light and shade of varying length for the plants (these periods can be more or less controlled depending on the technology used, as we will discuss later). However, not all plants are equally sensitive to shade. For example, some crops do not tolerate alternating irradiation very well, and it is relatively difficult to predict the productivity of crops subjected to alternating light and shade. The effects can then be quite severe, with, for example, physiological disorders on flowering and fruit set (especially when flower induction is important) and shifts in maturity dates. Others, on the other hand, such as the so-called “mid-shade” plants, accept these different periods rather well (products originating from the undergrowth such as small fruits and kiwis, leafy vegetables such as lamb’s lettuce or chard, etc.). Some trees, for example, have leaves that fall later because the environment under the panels is less stressful for them.
Note that many plants will be able to adapt to shade phenomena: leaf enlargement, changes in the spatial distribution of leaves on the plant, changes in the concentration of chlorophyll and carotenoids, etiolation and phototropism to seek light. We can “help” certain crops to adapt, for example by changing pruning practices in arboriculture to play on the alternation of light, or on the contrary, preferring crops that we know can better withstand shade. Photovoltaic panels, especially when they are fixed, can lead to heterogeneous light under the panels. While some operators propose to compensate for these effects with controllable technologies, other operators will propose different panel arrangements (in strips or in a checkerboard pattern – we will talk about this a little later) or will adapt their cultivation itineraries accordingly (alternating and/or differentiated sowing according to the zones under the panels, installation of a white mulch on the ground, installation of diffusing sheets on the walls and the roof. Could we even imagine managing the grassing of the inter-rows (and not only the crops) with shade control? In any case, if we were already having trouble explaining production variability within a plot, adding an additional heterogeneity variable (light or shade) will not make our task any easier.
As mentioned at the beginning of this paragraph, shading can indirectly have a positive effect on plant evapotranspiration when the temperature of the canopy decreases, with a consequent reduction in the need for irrigation (some projects involving photovoltaic tables over irrigation canals are also underway to limit water loss through evaporation). In dry areas, photovoltaic panels can indeed contribute to the reduction of evapotranspiration. When there is no water stress, however, the panels could be an obstacle to photosynthesis. More generally, if we look at water use efficiency, i.e. the ratio between the relative variation of dry matter and actual evapotranspiration, it is clear that it does not improve systematically – it all depends on the climatic conditions in the environment.
Continuing on the subject of water, the use of photovoltaic tables can raise questions about the spatial heterogeneity of water under the modules, but also about the heterogeneity of water in the soil. Photovoltaic arrays can indeed generate gully or water curtain effects at the edge of the panel (especially when the water is not collected in dedicated gutters). It could be relevant to take a more serious look at the soil, both in terms of the effects of erosion around the panels (even though some studies have found that erosion was less under the panels because the soil was more humid because it was less directly exposed to the sun’s rays), but also in terms of the creation of micro-climates in the soil, or the effect on soil structure and hydromorphy. Some experiments also seem to show that soils in the shade do not warm up or mineralise in the same way. This is something to be investigated…
By protecting from rain, photovoltaic tables could have a beneficial effect on the development of diseases and fungi (one can think of oidium and mildew for example) since the humidity and hygrometry conditions are completely changed. This aspect could be interesting for farmers wishing to move towards organic farming in the sense that limiting the development of diseases could help them to do without phytosanitary products.
Protection against climatic hazards
Photovoltaic tables provide significant protection against the vagaries of the weather. Against frost, the panels placed horizontally can act as infrared reflectors and reconstruct a partial greenhouse effect on the roofs. Radiative frosts can occur in early spring. On a clear night, radiation from the ground is rapid and high and can cause plants to lose a lot of heat – this is called white frost. Also note that within a few degrees, a flowering can be completely lost. Against hail, the panels can be positioned vertically, with anti-hail nets hanging down, or horizontally, so that the hail does not hit the plants directly. Against excess heat or light, the panels – if controlled – can limit the amount of sunlight on the crops (these shading effects can also be interesting to improve the working conditions in the field). Some territories prefer to whitewash greenhouses or nets to offset these effects (it is up to the reader to question this practice or not). This effect of protection against the heat may nevertheless require adapting the cultivation operations as well. For example, fruit under photovoltaic tables would be much more sensitive to the sun, as they are less adapted, and could suffer from burning if put in the sun (some farmers will have had to stop putting their harvest boxes outside).
During heavy rainfall, the panels – positioned vertically – will seek to limit the effects of run-off and water curtains on the plots. Photovoltaic infrastructures can be used as supporting structures for other climate protection tools. Examples include hail nets or rain covers. The structures can also support irrigation networks with water collection gutters at the edge of the tables. The effect of the panels on the wind – as obstacles or bottlenecks – has not yet been widely studied. However, the farmers on the estate I visited would have reported an earlier arrival of aphids under the panels, which would have seen them as an interesting shelter from the wind.
Photovoltaics and agricultural production
In terms of quantity of production, the effects of photovoltaic installations seem to be neutral to negative overall. In any case, plants would not need 100% light to grow at their best. Some plants would even see their growth stimulated rather for average levels of shading. However, it is when reaching high light obscuration levels that a significant loss of yield could be expected.
In any case, the relationship between the decrease in light radiation and yield is not linear. Some recent projects have nevertheless shown an increase in productivity under the panels with very fine control of the light (by limiting the periods of photo-inhibition during intense sunlight so that the plants do not stop growing in the middle of the day). The long-term effects of this have not yet been studied, but should not be overlooked. By limiting photosynthesis during the season on a perennial plant, there is a risk of affecting the following year’s development (reserves are affected, as is floral induction). The hypothesis of the light saturation point is worked out at the leaf level. At the plant scale, the question is perhaps different in that the light arriving on a leaf will be transmitted and diffused to the other leaves of the plant. Energy flow issues change scale, and perhaps affect the way we think about light and shade effects on the plant. In view of the large inter-annual climate variability, long time series seem necessary to tackle the problem. However, the questions are complex: how can we isolate the influencing factors over the years, especially for perennial crops?
Unlike yield, which remains a relatively sober parameter – both to describe and to measure – crop quality considerations are much more numerous as a result of the impact of photovoltaic tables on shade and canopy temperature. Sugar levels (and therefore alcohol levels for the vines) could be reduced, something that could be overcome by initially choosing sweeter varieties. Taking a step back, this could, for example, be an opportunity for arboriculturists and winegrowers to have a wider range of harvesting choices (the tables could make it possible to extend the vine cycle and shift ripening back to September, rather than August, with shorter, cooler nights beneficial to anthocyanins, tannins and polyphenols), by being able to play notably on the steering of the photovoltaic tables and on their choice of harvesting dates. Fruit and vegetables could also tend to be less colourful (the choice of varieties – this time more colourful – could once again be a relevant palliative). Agri-voltaic operators are more likely to put forward logistical arguments. By protecting the fruit from potential defects (cracking, burning, deformities….), PV systems could avoid problems with grading, quality level changes, or even pushing back certain fruits – and thus not negatively affect farmers’ marketing strategies. However, the quality and organoleptic parameters are much broader than that: starch content in fruit, fruit firmness, anti-oxidant levels, protein content in fodder; the list could be long…
Animal production
Although more recent, farm agrivoltaism is tested in a wide range of animal productions, and it is mainly animal welfare that is put forward. The animals (cattle, sheep, poultry, fish, bees, etc.) mainly benefit from the shading offered by the photovoltaic tables, which makes it possible to reduce the air or water temperature (in the case of fish ponds) and thus reduce the animals’ body temperature. In committing to welfare, the entire photovoltaic installation must be adapted to the presence of animals: raising the structures so that the animals can move around and not have to crawl under the panels, protecting the electrical cables, protecting the sharp edges, etc. Some welfare issues remain unresolved. The low table height thresholds, often of the order of one metre in the main existing documents (IDELE, Ministry decrees), seem insufficient in view of the animal dynamics under the panels (what about the overlapping of a ewe by a ram around a photovoltaic table barely 1 metre high?). Electromagnetic fields (particularly at the level of the inverters which produce the most) could have an impact (level of production, disturbance of milk quality, stress, etc.) on animals present for long periods near the panels (even if rotational grazing could help to avoid the problem). More broadly, the consequences of the arrival of an agrivoltaism power plant on the entire breeding system should be investigated (consequences for the farmer, complexity of surface management, etc.). Some projects also put forward the grazing of animals (especially sheep) for plant production under panels. While these systems are commendable, some may be criticised for imagining putting halters on the animals to prevent them from raising their heads too much and concentrating on what is on the ground rather than the trees…
In the context of livestock production, the argument of adaptation to climate risk is a bit more difficult to justify. The real benefit of photovoltaic tables in livestock production remains shading, but one could consider that this benefit could just as easily be provided by trees. For grassland growth, shading may indeed be relevant, but it would be necessary to be able to measure the biomass gain at the scale of the whole farming system. The argument of photovoltaics as a way of securing the autonomy of fodder in suckler herds, in the sense that panels would be placed everywhere to ensure the productivity of the meadows, remains rather limited. As for the main agricultural productions we have discussed, the impact of shade on the growth of grasslands is still relatively unknown, as is the choice of the most suitable varieties to compensate for the presence of photovoltaic panels. Nevertheless, the photovoltaic tables seem to be interesting for ensuring a more even grassland production over the year. In spring, the shoots under the panels, protected from the sun’s rays, develop less than their neighbours. On the contrary, during the summer, when the sun is at its maximum and the grassland not protected by the photovoltaic tables suffers from burning and heat stress, the grassland under the panels tends to have a much more pleasant time.
Surprisingly, it seems that the majority of studies consider the influence of photovoltaic tables under panels to be all things being equal. In livestock farming, however, interaction with animals is permanent: sheep can graze, trample, pack or rest on the cover under the panels. The isolated effects of the panels that we have described above could thus be counterbalanced by animal disturbances. The value of grassland production under panels could also be questioned in the sense that, as the animals spend a large part of their day under the panels, the quality of the soil is still much more degraded there than elsewhere.
It seems that most photovoltaic projects are not yet thought through in a systemic way for animal production. They are perhaps still too many classic installations, with low panels whose design does not meet the needs of agricultural activity (it is even conceivable that panels that are too low could also be hampered in the long term by the excessive growth of a meadow). It is perhaps necessary to keep reminding people that eco-patterning is not an agricultural production. The IDELE guide on ruminants in an agri-voltaic context clearly shows the agronomic considerations that need to be taken into account when setting up such installations, including the management of livestock equipment, the design of power plants, the choice of cover, the choice of grazing systems (rotational or continuous), the type of animals (empty, pregnant, suckling females – with animals potentially in a period of struggle), and the ergonomics of the farmer’s work (IDELE, 2020). In addition, mixed grazing systems, mixing sheep and cattle, could be relevant because the two species are complementary in their grass harvesting. This cohabitation could reduce the number of refusals by the animals and ensure better management of parasitism.
Can we measure the synergy between agricultural and electrical production?
Is it possible to objectively assess the benefits of installing a photovoltaic system on a farm? From a purely financial point of view, the answer is yes, and even twice yes.
- The LCOE (Levelized Cost Of Energy) is calculated simply as the average price per kilowatt hour over the lifetime of the PV plant (a lifetime of 30 years is generally used as a reference threshold). International LCOEs are around 30-45€ per MWh. Grassland PV plants (livestock agrivoltaism) might even have some advantages over standard plants because there would be less land preparation work and lower land and soil maintenance costs. Greenhouses and shadehouses would have higher LCOE than PV plants on agricultural land (mainly due to the more expensive PV modules and possible trackers installed).
- The ROI (Return on Investment) is the number of years during which electricity production must be ensured to make the installation of the plant profitable. In an agricultural context, the ROI is unsurprisingly higher than in a conventional context (some players have told me that it is a factor of 2), notably because of the dust that can be found on the panels following agricultural operations close to the photovoltaic tables or the passage of animals, the higher humidity due to the use of phytosanitary products, or the corrosion potentially induced by the cultivation operations.
From an energy point of view, it will be preferable to use EROI (Energy Return On Investment) rather than ROI (you may also hear it referred to as ERR). The reasoning is very similar; the difference is that instead of talking about monetary aspects, the question here is when the photovoltaic installation will have produced the energy that it took to build and install the photovoltaic plant project. The EROI is sometimes presented more as a ratio between the usable energy (in our case by the panels) and the energy consumed to obtain it. It is often the EROI indicator that is used to compare the different existing energy sources.
In adding the agri-voltaic dimension, some authors have preferred to turn to an indicator used historically in agro-forestry to compare the performance of the association of two different crops with that of the same species grown separately: the LER (Land Equivalent Ratio). This indicator is defined as the relative area needed for pure crops to have the same production as the combination of these crops. An LER greater than 1 indicates a better performance of the crop combination than of the pure crop. In an agrivoltaic context, the association is not surprisingly considered between a crop under a panel and the production of photovoltaic energy. If the shade of the panels and the installation of the photovoltaic structure had no influence on the agricultural production under the panels, the LER indicator would take a value of 2, since on the same agricultural area, as much can be produced (either in terms of plant yield or electrical energy via the panels) as if the panels and the crop were geographically separated. As photovoltaic panels are not totally neutral on agricultural production, the LER tends to oscillate between values of 1 and 2, depending on whether one degrades the photovoltaic value (reduced module density, panel fading) or the agricultural value (full module density..). The LER will never be less than 1 since even without any agricultural production under the panels (the panels are for example completely covering the crops), there will always be electrical production with the panels. However, some stakeholders advise against using the LER indicator because it would not distinguish between PV projects that erase part of their solar output for the benefit of crops and those that degrade the same proportion of agricultural yield.
Rather than focusing on light, we could, for example, consider water use efficiency (WUE) as a way of looking at the services provided to agriculture.
Perhaps we could also focus exclusively on energy and compare the electrical energy produced by the panels with the energy contained in the agricultural production at harvest (in calories)? The energy conversion rate of the panels, i.e. the difference between the energy produced and the energy received by the sun (currently around 15 to 20%) would be much higher than the conversion rate of photosynthesis (more like a few percent).
The Commission de Régulation de l’Energie’s calls for tender and private contracts
Agrivoltaism projects are processed through two distinct branches
CASE N°1 : the project is submitted in response to a call for tenders (AO) from the Commission de Régulation de l’Energie (CRE); this is known as an AO CRE. Every year, the CRE opens a certain number of calls for tenders that allow access to a guaranteed feed-in tariff for electricity. These calls for tender are of two kinds:
- An innovation call for tenders (AO CRE Inno) for which the agrivoltaic project must demonstrate the implementation of an innovative device (whether technical or agricultural). These calls for tender involve projects that are relatively limited in terms of photovoltaic production (the maximum unit power threshold is 3MWp). The CRE innovation calls were set up to boost the sector (with very attractive electricity buy-back prices – over €100 per MW – and we agree that it is always the taxpayer who pays out of his pocket…). Eventually, the CRE innovation calls should disappear when the market is mature enough. As photovoltaic technologies are deployed (and mature) in the field, it will be increasingly difficult to justify the innovative nature of photovoltaic installations. With total innovation tenders of 70MWp over the year, we can expect about thirty projects (since the only maximum per project is 3MWp). It is difficult to imagine that each of these projects really contains an innovation (and some projects that claim to be innovative could be widely questioned). Generally speaking, these calls for tender are mainly used for projects with high added value crops. Important information: the CRE has recently opened these calls for tenders for innovation on livestock agri-voltaism projects.
- A more traditional call for tenders (AO CRE) which, if the project is accepted, commits the photovoltaic operator to pay collective agricultural compensation to the farmer who will see a photovoltaic infrastructure installed. This collective agricultural compensation is quite similar to environmental compensation in its reasoning. The idea is to compensate for the significant erosion of agricultural land and to take into account the individual impacts of photovoltaic projects on farms (payment of land compensation to the owner and eviction compensation to the farmer). This compensation can take several forms: investment aid, promotion of agricultural products, development of new markets, aid for professional training or improvement of local infrastructures. The higher the compensation, the lower the quality of the agri-tourism project. These CRE tenders are mainly used for ground-mounted photovoltaic plants. It is possible to go for large areas and large power installations. The market would tend to direct agrivoltaic power plant projects for which the panels are at least 1.80 m from the ground towards roofing calls for tender and power plant projects at more basic heights towards calls for tender for ground-based power plants
Operators who go through CRE negotiate their feed-in tariff with CRE; one could say that they apply for a feed-in price when they submit their project (the carbon footprint of the panels is obviously counted in the final score of the project). Operators who win classic CRE tenders can also benefit from a remuneration supplement (which has nothing to do with the collective agricultural compensation of the CRE tenders, and which also has nothing to do with EDF’s purchase obligation contracts for electricity, which are only suitable for photovoltaic installations on buildings). This remuneration supplement is aimed at producers who sell their energy directly on the markets. Energy producers receive a premium that compensates for the difference between the income from sales and a reference level of remuneration, set individually for each installation. This remuneration supplement is awarded after a competitive bidding process for photovoltaic installations with a capacity of more than 500 kWp (the threshold was raised in 2022 – it was previously available for capacities of more than 250 kWp; i.e. many more installations). The CRE calls for tenders give the Ministry of Ecological Transition the possibility of sanctioning projects that no longer comply with the criteria of the calls for tenders
The CRE distinguishes between photovoltaic projects on agricultural land and agrivoltaic projects. Note that agri-voltaic projects do not have any dedicated call for tenders for the installation of photovoltaic power plants, with the exception of the CRE Innovation calls. Calls for tenders for installations on agricultural land were opened in the second half of 2021 for all agricultural areas of municipalities subject to national planning regulations and communal maps. However, installations on municipalities subject to local urban plans are not eligible, which is the majority of municipalities (Figure 5). For the installation of photovoltaic infrastructures on degraded land, the CRE regularly issues more specific calls for tender.
CASE N°2 : the project does not fit into the CRE’s calls for tender. The photovoltaic operators then negotiate a private contract on the market with the customers to whom they will sell their electricity. This is known as a PPA (Power Purchase Agreement). As you may have understood, there is no state guarantee or subsidy for this type of contract. The photovoltaic operator rents the electricity grid (and becomes an electricity supplier) and thus sells its electricity to its customers. These contracts are often long term (5, 10 or even 15 years), rather with industrialists who will commit to buy electricity, mainly to decarbonise their daily activities. These photovoltaic power plant projects are generally installed on much larger surfaces since, as there is no guaranteed feed-in tariff and the feed-in prices are often lower than those of the CRE (around 50€ per MGW for PPAs against 60-70 per MGW for CRE calls and up to 110-120€ per MGW for CRE innovation calls), the operators are looking for economies of scale. Unsurprisingly, the big challenge remains the price of electricity. It is important to understand that photovoltaic installations on agricultural land can also be developed via private contracts, and not only within the framework of CRE calls for tender.
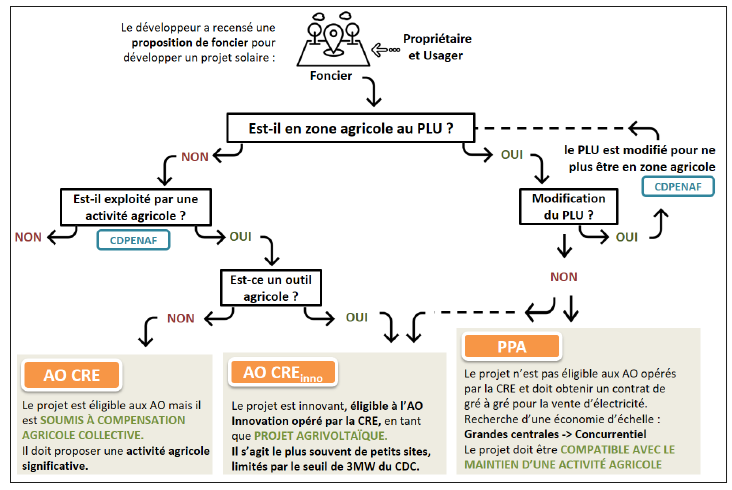
Figure 5. CRE tenders and PPAs. Source: ADEME (2021).
Note that changes to the CRE are on the agenda to meet the objectives of the multi-annual energy programmes (MPE), the question being whether it is preferable – in the agri-voltaic context – to set up large power plants near the electricity distribution nodes or rather small plants that can be easily connected to the grid. In the case of large plants, deployment would be more time-consuming (creation of files, carrying out of environmental studies, and could require significant investments from farmers). Small plants, on the other hand, would be easier to deploy and could reach many farmers as there would naturally be more plants. The risk for these small plants is that they are poorly designed and built in a hurry.
The economic models of the agrivoltaic sector
A wide variety of players and business models
Agri-voltaic power plant projects involve several types of actors. There are :
- the farmer who will have the photovoltaic installation above his production.
- The agrivoltaic operator who carries and develops the power plant project.
- The landowner, who is the owner of the agricultural land on which the power plant will be built.
- A service company – especially in the case of dynamically controlled photovoltaic panels – which has developed the technologies and algorithms for controlling the panels.
The economic models are complicated by the fact that the roles of these actors are sometimes a little mixed. The landowner and the farmer may be the same person or they may be two different people (some PV operators still do not distinguish between a landowner and a farmer…). The PV operator can be the landowner (although this is rarer). And the service company is not yet present in many different business models. With this set of actors, the business models of agri-voltaic projects are quite varied. One can find models where :
- The photovoltaic operator pays a rent to the farmer (more or less important amount) to rent the agricultural land on which the operator installs the photovoltaic plant
- The farmer self-finances his project. If the farmer is not able to provide the necessary equity from his bank, he can be a minority or majority shareholder in a project (with participatory financing from citizens or the territory). Photovoltaic projects can be launched by a collective of farmers
- The landowner establishes an emphyteutic lease (more or less important amount) with the photovoltaic operator
- The photovoltaic operator finances the photovoltaic infrastructure for the farmer (e.g. a greenhouse on which photovoltaic panels will be placed). It is therefore the PV operator who invests (for the greenhouse in this example) and operates the PV panels. This format does not cost anything to the farmer but it is the photovoltaic operator who is the owner of the whole. In some cases, the operator may also own the plant material.
- The service company sells a dynamic control service to the farmer and a patented technology to the PV operator (the service company contracts a pre-calculated clearing rate of the PV tables and guarantees to the PV operator that the tables will not be cleared all the time). In this case, it is important that the service provider is not a company that is too close to the PV operator to avoid any conflict of interest.
These business models can, to a greater or lesser extent, be combined. The PV operator is of course remunerated from the sale of the electricity produced by the plant.
The loss of CAP aid
Surprisingly enough, farmers who engage in agrivoltaism lose their rights to CAP subsidies. For those who have made sure to keep a strong agricultural activity under the photovoltaic tables, the shower is cold. What kind of aid would it be? It would seem that we are talking about basic payment entitlements which represent a substantial sum for certain farms. If we were to be fussy, we could at the very least remove the space taken up by the panels (with a comfortable margin) and also consider all the surfaces linked to the installation of a power plant (fences, paths, inverters, etc.). Moreover, as the power plants are considered as industrial sites, the fire brigade often imposes limestone paths to be able to access the land (and thus indirectly to destroy some extra soil.) Even if an inverter were to catch fire, is there a risk that it would spread to the whole power plant? If agri-voltaism is considered as a device at the service of agricultural production (and we have seen that the potential services are numerous – both agronomic but also by offering the farmer the possibility of reinvesting in his farm), penalising the farmer economically by excluding him from CAP subsidies seems out of touch with reality. However, some agricultural actors would see behind the reduction of CAP subsidies a way to increase the amounts of collective agricultural compensation.
The payment of a rent: a risk for the agricultural sector?
The most worrying issue is still the remuneration of the farmer. The photovoltaic and agricultural worlds do not necessarily understand each other. The average gross margin per hectare of a farmer is close to 500€ (perhaps excluding the peaks for rape and wheat). By sometimes offering farmers rents of several thousand euros per hectare for the installation of agricultural projects, photovoltaic operators do not seem to be aware of the issues and problems of the agricultural world. Several players are also against such schemes and are not in favour of a model with a rent to be paid to the farmer. For some, paying a rent would be tantamount to calling into question the sustainability of the agricultural system.
The introduction of a rent could lead to a risk of agricultural abandonment (downgrading of the agricultural character of the land in the urban planning regulations, marked degradation of agricultural production), competition to take over farming if the land is no longer accessible to young farmers, or inflation and destabilisation of agricultural land, with land prices likely to soar. In addition to land speculation linked to electricity feed-in tariffs and the margins of the photovoltaic sector, some operators tend to adopt aggressive land strategies with a desire to retain and secure land (in order to avoid having their land stolen by competitors). Some operators will even go so far as to propose very high rents, simply to push farmers to sign contracts, which the operators will then sell to other developers looking for land. Developers will sometimes be prepared to pay very high prices for these contracts to secure land. Other business providers will secure land where it is not always technically feasible with farmers who will find themselves stuck with promises of leases that cannot be overly valued. Other operators, on the contrary, will sign these lease contracts with conditions precedent (for example, only if a building permit is signed). Others will wait for good economic conditions to develop before committing themselves.
Suspending the rent paid would then be a way of ensuring that the sharing of value is really considered from the point of view of the service provided to agriculture (adaptation to climate change, protection against hazards, etc.). It is nevertheless difficult to blame farmers who would accept such amounts when we see the salaries of certain parts of the agricultural sector. In precarious conditions, or when a farmer cannot find a buyer, it is understandable that he would accept a high remuneration for the installation of photovoltaic projects.
The farmer’s remuneration on these agrivoltaic projects must not exceed 50% of the farm’s turnover in any case, at the risk of the farmer’s status being modified. Some farmers will nevertheless have thought of dissociating the profit from agricultural production and the profit from photovoltaic production in order to be able to collect a nice pactol (we will talk about this later with the photovoltaic project companies). The fact remains that the rent for the farmer, if it is paid, must remain relatively low so as not to disrupt the agricultural occupation. The purpose of this rent would be to compensate for the loss of CAP subsidies (even if this laudable objective does not address the real causes of the issue), to improve the farmer’s living conditions, to reward the risk taken by investing in photovoltaic tables (loss of yield, loss of profitability, etc.), and to support the farm in possible strategic investments insofar as this rent provides a stable, long-term income for the farmer. This rent can thus, without becoming preponderant, be a pleasant support and a cushion for the farmer. The rent has to be fixed on a case-by-case basis, depending on the type of farmer, the surface of his land, or the type of profitability that the owner of the land had (for example, he may no longer have the means to ensure an economic activity on his agricultural land).
The photovoltaic operators are also trying to make a go of it. Agri-voltaic projects cost between 800,000 and 1 million euros per hectare. Conventional power plants are less expensive, but the agrivoltaic dimensioning requires the addition of supporting structures for the photovoltaic tables (piles, etc.), notably in iron and steel. With the increase in the price of steel, and the cost of transporting panels, mainly from China, costs continue to rise. And photovoltaic operators will be looking for a minimum surface area to install power plants in order to achieve a certain profitability. The competition is tough and the economic context is not always obvious. With the increase in the cost of equipment and the decrease in the electricity sales tariffs in the tenders, it is no longer always interesting for the PV operator to pay for the equipment.
As each photovoltaic operator has its own economic model with its own remuneration levels and contracts, it is not always easy to compare offers. However, it is often in the other aspects that operators can distinguish themselves: commitment to agricultural activity, alignment between the photovoltaic project and the agricultural project, participation in the project’s capital, participation in preventive maintenance operations, agricultural technical support (switch to organic farming, HVE, etc.). Some will recommend simpler methods such as charters or commitments to be signed as an annex to the power plant projects.
Photovoltaic operators are not necessarily closed to the establishment of a floor and ceiling price for rents (to the landowner and/or to the farmer) that serve as an incentive for the farmer to maintain an agricultural activity. Some actors propose, for example, to provide no more than a farm rent to the landowner (bearing in mind, however, that some landowners may be former farmers with meagre pensions). The interest for the developer is mainly one of image, to ensure the acceptability of their projects by the actors of the territory. Theoretically, one could of course think of cutting the farmers’ remuneration if they stop agricultural production under the panels but, from a regulatory point of view, this is not really allowed. And it would be all the more complicated for an owner to implement. It is possible to imagine quite sophisticated economic models in which a farmer would operate a photovoltaic project for several years and then pass on his activity to another farmer. In this transfer, the owner also has a say and may want to intervene.
Insurers are also starting to get back into the game by seeing photovoltaic tables as a tool for managing climate risk. Climate insurance in agriculture is undergoing a major reform (I refer you to the corresponding blog post for interested readers), and insurers and reinsurers have an even greater interest in positioning themselves as the costs of climate-related disasters continue to rise. In the photovoltaic field, insurance systems can, for example, intervene to insure against a lack or deficit of sunlight with dedicated parametric insurance schemes.
What type of contract should I choose? Farming, Commodat, Emphyteutic Lease? If the status of fermage seems to be forbidden in view of the commercial nature of photovoltaic activities, the two other types of contracts seem to be the most appreciated between landowners and photovoltaic operators. The signing of a lease agreement is nevertheless very binding for the landowner. The commodat – as a more flexible (but also more precarious) contract – may be preferred. Long-term contractualisation remains an issue for farmers and landowners to ensure that they are sufficiently covered. Could we imagine agrivoltaic rural leases?
Generally speaking, the impacts of photovoltaic projects on the economy of farms as a whole are relatively little mentioned, and perhaps not sufficiently addressed. The subjects to be considered are extremely numerous:
- Possible loss of CAP support,
- potential rents from the plants,
- impacts on agricultural production in terms of quantity and quality,
- organisational, operational and logistical changes in agricultural itineraries (tree thinning costs can be reduced, hail net costs can be reduced because the supporting structure is there)
- work rates can be increased if plant designs are not sufficiently thought through, potential need for equipment renewal…),
- salary issues (with potential additional salary requirements as a consequence of the installation of a PV project) or
- energy related issues (what about saving money on lighting and using the heat produced for self-consumption versus the cost of the lighting system, especially in the context of rising energy costs)
- ….
In short, there is plenty to ponder!
Towards ever more outsourced power plant projects?
PV operators do not necessarily manage a project from start to finish. Some developers actually only prospect and then sell the projects once they have secured them. The PV operator will then call on service providers to build the installation on site (in France, very few companies build power plants – understand that this construction is therefore often outsourced by the PV operators). Other operators build and sell. Others take care of the operation until dismantling, which gives them the advantage of evaluating the project in its entirety and complexity. And this is sometimes also an advantage for the farmer. With projects being bought or exchanged from hand to hand, it is not always easy to know who to turn to when there is a problem. Between an operator close to the farmer and someone at the other end of the world on the other end of the phone, the context is not really the same.
The maintenance and repair of power plants are mainly managed by the photovoltaic operators. They can sometimes be criticised for organising repair routes and possibilities that suit them, and which are not always well thought out with the farmers. Entry to the plants, which are often fenced, requires accreditation and training in electricity (farmers also need to be trained). On the animal production side, farmers are often asked to maintain the vegetation around the photovoltaic tables in their contracts (to avoid the development of shrubby species, clearing of undergrowth…). If farmers are much more often present than operators in the power plants – as farmers are obliged to do – the supervision of the power plant is also often their responsibility, and this time should not be underestimated: monitoring the electrical production of the installation, both instantaneously and over time, alerting the operator in case of production stoppage, checking for micro outages and shading, and comparing the inverters when there are several.
Technological and digital tools around photovoltaic panels
Fixed and dynamic agrivoltaisms
When discovering the field of agri-voltaism, one can be quickly surprised by the technological profusion at work by developers to make agricultural and energy production cohabit. These technological advances are of several kinds:
- The height of the photovoltaic tables in relation to the ground the necessary spacing between the tables
The minimum height between the ground and the photovoltaic table is generally referred to as the “bottom of the table” height (tables are often tilted – the bottom edge of the table, closest to the ground, is then considered). The lower and more fixed the tables, the cheaper the total infrastructure. Some people may have the image of very low, large-area (non-agricultural) ground-mounted plants that are really more like fields of mirrors than anything else. From an agronomic point of view, one would tend to opt for higher structures. One of the main advantages of setting up high structures is to facilitate cultivation operations and the passage of machinery under the panels. If the structures have to be raised, the price of the structures increases, as it requires a more solid composition of the infrastructure (using steel and increasing the number of posts to hold the tables) and anchoring the structure to the ground (sometimes using concrete) to avoid mechanical problems and the risk of being torn off by the wind (the structure is much more sensitive to the wind the higher it is above the ground – it is like a sort of sail or kite effect). Some operators propose high structures, with photovoltaic tables held in place by cables and wires, an interesting way of limiting the ground area of photovoltaic plants by reducing the number of poles and piles. However, the height of the panels is always a matter of compromise. In addition to the operational issues of machine passage for farmers, panels close to the ground will tend to induce sunshine and heterogeneous water distribution under the panels, whereas panels at a height risk generating edge effects with light rays penetrating under the panels by the edge of the plot.
In direct relation with the height of the photovoltaic tables, the spacing between the tables is also important to ensure synergy between agricultural and photovoltaic approaches, both to maintain cultivation operations and machine passages but also to facilitate the movement of animals between the panels. This is referred to as the coverage rate of the photovoltaic tables on the plots, a ratio between the horizontal surface of the panels and the surface of the agricultural land under the panels. Wide rows between the photovoltaic tables make it possible, for example, to harvest hay, to shred animal waste (which has not been grazed) or to weed. The spacing between the tables also raises the question of the number of poles or piles used to hold the structures. With so-called bi-pile structures (two piles to hold each table), cultivation operations are necessarily made more difficult than with single-pile structures.
- The density of photovoltaic cells
Not surprisingly, the density of the photovoltaic cells on the tables will directly influence the amount of light reaching the plants under the panels. The optimal arrangement and layout of the photovoltaic modules must be carefully considered to limit and/or distribute the shading effects. Figure 6 shows a sample of possible arrangements of modules above a photovoltaic greenhouse (single or double strips, staggered or checkerboard strips, low or very high density, alternating or glued strips…). Many agricultural actors still have in their memories the images of photovoltaic greenhouses completely covered with fixed shading that offered almost no luminosity for the plant (we will talk about this a little later).
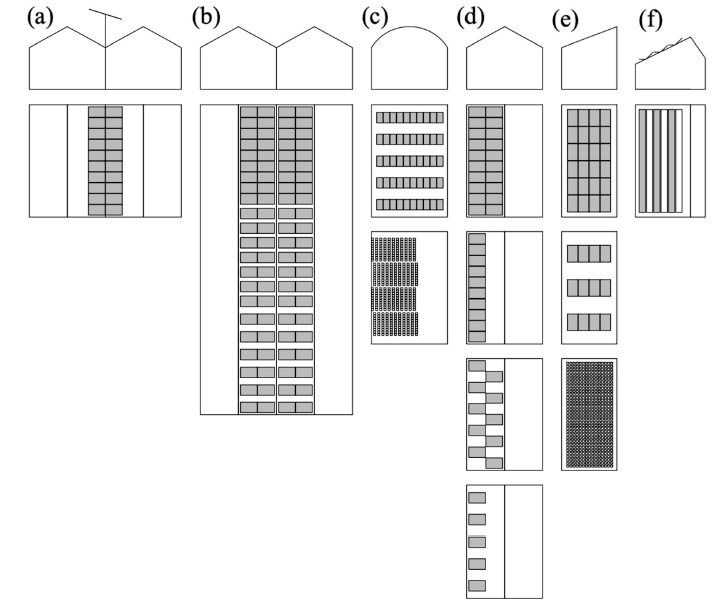
Figure 6. Various arrangements of photovoltaic panels on a greenhouse
- Orientation and tilt of photovoltaic modules
Managing the orientation of the photovoltaic modules makes it possible to work on the shading of the plants. It is possible to find photovoltaic parks with tables oriented North/South or, on the contrary, East/West. Some parks are actually configured in such a way as to optimise the ratio between the surface area of the photovoltaic tables and the installed power of the plants. The more north-south oriented tables in the length support fields of panels oriented on one side to the east and on the other side to the west. In these structures, the density of the photovoltaic tables is often higher and these plants sometimes tend to limit the passage of light and rainwater runoff to the ground (unless translucent modules, dynamic steering, and spacing of the tables are used to allow water to pass more easily).
In plant systems where the inter-rows (or rows) are sufficiently wide, the panels can sometimes be placed vertically to allow the passage of agricultural machinery (a way of maintaining a form of agronomic rotation under surfaces of photovoltaic tables that are sometimes more difficult to access)
- Single-sided (mono-sided) or double-sided (bi-sided) panels
Bifacial photovoltaic panels, as their name implies, can capture solar radiation to produce electrical energy on both sides. The interest of these bi-facial modules in agriculture is to be able to capture the radiation reflected by the plant cover (via the albedo effect of the cover). This bi-faciality is also interesting in that it combines well with solar tracker systems, which no longer need to work on 180° angles (it is therefore possible to work with simpler tracker systems) since both sides of the panel are able to produce energy.
Power plants with single-sided and double-sided modules (not necessarily on the same power plants) are interesting to combine because they can ensure complementary power supply to the grid. Solar energy can be marketed at times when south-facing parks produce less and electricity prices are higher.
- Type of supporting structure : Fixed and dynamic structures
Photovoltaic structures can be fixed – the tables do not move and keep the same layout, location and orientation throughout their installed life – or mobile, i.e. in this case the tables have the possibility to move in several axes. This is commonly referred to as “dynamic” agrivoltaism. Photovoltaic installations can take several forms
o The tables can slide (a bit like opening or closing a veranda). These systems can be limited by the spacing between the poles and the crop rows
o Tables can change orientation on a single axis by following the path of the sun (this is called a single axis structure) with solar tracker systems
o The tables can change orientation on two axes following the path of the sun (this is called a bi-axis structure – the mobility is therefore greater than in the case of mono-axis) with solar tracker systems
The dynamic control of the tables via solar trackers is interesting to solve the problem of the spatial heterogeneity of the daily solar distribution for the crops (and of the temperature heterogeneity which can be higher under the fixed panels compared to control areas). It should be remembered here that in addition to the shading effects on crops, sunlight heterogeneity can also pose practical problems and affect the organisation of harvesting for farmers because not all crops will necessarily be at the same stage of maturity. Solar tracker systems are generally able to rotate the panels over angles of +/- 70° (some operators have been reported to go up to +/- 90° and cover a full 180° angle range).
Fixed technologies can accommodate greater slopes of terrain (and also allow for greater coverage of the terrain – with a greater density of tables and modules).
The use of mobile structures also offers the possibility of coordinating with agronomic objectives during climatic hazards:
- Horizontally, the tables shelter the crops from hail and also create a kind of greenhouse effect to protect from potential frost at night
- Vertically, the tables allow a better distribution of rainwater and avoid water curtains that will always run off in the same place on the ground
- If there is a risk of burning on the fruit during a very hot spell, the tables can protect the crops by maximising the amount of shade.
- In case of strong wind, it is necessary to be able to place the panels in a safe position because the photovoltaic structure can be very load bearing.
In the introduction, we mentioned the notion of the saturation point of light by the plants. This hypothesis, which is widely accepted by the energy and agricultural sectors, suggests that the excess light that cannot be used by the plant could be used to produce electrical energy via the photovoltaic panels. The concept of the saturation point may actually be a little more complicated. Plants would respond not only to light (for photosynthesis) but also to alternating light and shadow. Generally speaking, the photosynthetic apparatus of the plant is always excited and ready, as soon as it receives light, to function for the benefit of the plant. If the plant is in the shade, this photosynthetic apparatus is switched off and it will take some time for it to start working again. In shaded conditions, especially under fixed structures, the plant will tend to adapt to these conditions and may develop shade leaves. The plants will etiolate (the internodes will increase in size to seek light), the plants will have larger but thinner leaves with a destructured parenchyma (with holes) and a thinner leaf surface. The leaves will of course still have photosynthetic capacity, but this capacity will be more efficient at low light levels (the plant will be adapted to these conditions). On the other hand, the leaves will be much more sensitive to high irradiation, and the leaves will then be much less efficient than a normal leaf at photosynthesis at medium light levels. These shadow effects can lead to numerous physiological disorders which we have already discussed a little (on floral induction for example…)
The dynamic systems allow us to work on the strategy of fading the panels – understand here that the panels “fade” to let the light reach the plants. Continuous tracking throughout the day makes it possible to manage the fade periods. It is, for example, possible to be in fade in the morning and to return to fade in the early afternoon. Once again, it is all a question of compromise. Several experiments have shown that there are thresholds of shade below which plant photosynthesis is little or not at all affected (some authors recommend maximum thresholds of 60% shade). At what point should the tables let in light? How much can the profitability of an agri-voltaic project be degraded by a dynamic control system? These questions cannot be neglected, since behind every PV infrastructure there are banks and financiers.
With tracker systems in place, shading is immediately less of an issue and may tend to take a back seat. It is perhaps water that can be considered as a limiting factor.
However, dynamic control can go much further than simple solar monitoring by trackers. This control can in fact integrate agronomic models to consider at the same time the growth of the plants under the panels and optimise their sunshine. This control is obviously all the more complicated as the crop system under the panels is complex (diversified systems, complex rotations, etc.) and as it requires the construction of several plant growth models. The specific growth models make it possible to work on the energy balances (carbon and water balances) that will be affected by the presence of the photovoltaic panels: the evapotranspiration of the plants is likely to decrease under the panels, photosynthesis may be reduced, the leaves may be warmer, etc. And these dynamic systems make it possible to ensure that light penetrates as much as possible at the different phenological stages of the plant (fruiting, flowering, etc.)
Accurately modelling plant growth in order to integrate it into these dynamic control systems requires access to information both on the plant itself and on these environmental conditions. Some experimental sites will have set up a number of sensors to ensure this monitoring. For example, there will be sensors
- on the photovoltaic tables (inclinometers in particular) to control their orientation in real time
- weather stations to quantify the climatic state of the plot (air temperature, leaf temperature, rainfall, irradiation, wind, PAR sensors to measure photosynthetic active radiation, leaf wetting sensor), to build plant evapotranspiration models and light interception models, and to be able to control the fading of the panels in the event of hail or excessive rain
- probes (tensiometric, capacitive, neutron tubes…) to monitor the water content in the soil, dendrometers and sap flow sensors to monitor plant transpiration and water status
- Video cameras for panel monitoring
- RGB, Multispectral, and Hyperspectral cameras to characterise the functioning of the plants and monitor their development during vegetative growth.
In short, some experimental sites will be fully equipped with sensors. A whole range of agro-pedo-climatic monitoring (soil analysis, maturity monitoring, etc.) may also be added to complement the agronomic models.
Tools and technologies under development
The technologies around photovoltaic panels are constantly evolving. These technologies are obviously not specific to agrivoltaism as such – some research is focused on improving the efficiency of photovoltaic panels – but it is always interesting to look at the trends. Here are some examples:
- Improved photovoltaic array structures
Some operators have started to deploy modular arches (i.e. 3-dimensional photovoltaic tables) that can be moved, and which integrate photovoltaic modules directly into their structure. We’ve already mentioned this briefly, but to make up for the heavy installation of piles and ground anchoring structures, some developers are putting up high tables of PV held up by cables and wires.
Mobile solar units are also beginning to emerge. These units can be thought of as solar panels pre-assembled in a container – the container consists of 200 pre-assembled and pre-wired photovoltaic panels. The beauty of these units is that they can be deployed and moved quickly. Although these units were initially designed for disaster areas (cyclones, conflicts, etc.), could they be used in complex crop rotations or fallow systems?
- Semi-transparent tables
Unlike conventional opaque tables, semi-transparent tables contain conventional photovoltaic cells but also transparent spaces on the table to let more light through. These tables, by construction, generate less power since there are fewer photovoltaic cells per square meter. However, the price of these tables is relatively higher than for conventional tables – so there is a trade-off between agricultural production and energy production by the panels.
- Cell improvement
Several research projects are underway to improve the energy yield of modules. The CEA is reportedly trying to achieve light absorption rates of over 45% (current commercial technologies achieve around 20-25%). It is also possible to hear talk of heterojunction (in reference to the NP junction in figure 2) as a technology different from current cells (the cells would be juxtaposed in different ways and the silicon typologies used would be doped differently) and which has the interest of having high efficiencies, and better bi-faciality coefficients. Current photovoltaic cells are mainly composed of silicon – these are known as “first generation” cells. Second-generation cells are called “thin-film” cells (their absorption area is only a few micrometres thick) and are said to be more efficient and flexible than their first-generation cousins. These second-generation photovoltaic cells include amorphous silicon, CdTe (cadmium telluride), CIGS (copper, indium, gallium and selenium) and CZTS (copper zinc tin sulphide).
- 3rd generation photovoltaic cells
These organic semiconductors or dye-sensitized solar cells could be interesting in that they could share the solar spectrum by producing electrical energy outside the spectral regions necessary for plant photosynthesis (the red and blue spectral bands of the visible spectrum in particular). They could be considered as photo selective covers. These cells could be particularly well suited to existing infrastructures such as greenhouses, where they could, for example, be glued to the wall. In spite of characteristics that seem promising in terms of flexibility, lightness, colour diversity, degree of transparency and environmental costs, these technologies are not yet stable or efficient enough to convert solar energy into electrical energy.
- Concentrator cells
The technology of luminescent solar concentrators (such as those developed by the Swiss company Insolight) seeks to concentrate a beam of light on a given point of a photovoltaic cell, thanks to optical lenses, to maximise its intensity. The interest of these concentrating cells would also lie in their ability to separate direct light from diffuse light (the latter being able to be transmitted to the crops). The cells would be able to follow the sun by moving horizontally a few millimetres a day to keep the cells aligned with the light beam component. These technologies are still in the development stage, are relatively expensive, and are relatively complex to implement given the precision required in moving the cells to ensure alignment with the light beam.
- Decision support tools
Decision support tools (DSTs) – of varying degrees of complexity – are also emerging to support agri-voltaic projects. These private tools are mainly web-based services; for example, there are DSTs for calculating the cost of an agricultural lease, for estimating the working time in the field with an existing agrivoltaic infrastructure, or tools for optimising the design of agrivoltaic power plants according to the climate and the agricultural activities in place.
- Agricultural robots under photovoltaic panels?
Since the environments under the panels are very structured and controlled, could we envisage having agricultural robots working under the panels (for example, weeding robots)? The fact that there is already an infrastructure in place could even be an asset for moving robots (on rails, or moving between poles like a chimpanzee…). The fences around the photovoltaic installations could be an opportunity to use robotic machines in a safe way, and not to be constrained by the Machinery Directive (which requires an operator to be present on site to ensure that the robot does not do anything). If we also consider the question of the energy autonomy of robots, the fact that these robotic units work under photovoltaic panels, i.e. with a potential renewable energy source at their disposal, could make it possible to move towards a reduction in the sector’s dependence on fossil fuels. The question of the interest and use of robots in agriculture is much broader than this very specific case study – we will discuss it in a future blog post. Patience, then…
An ontological framework to describe an agrivoltaic project
To go a little further in the description of a photovoltaic plant, I propose here an ontology (a data description model) for outdoor photovoltaic plants (excluding greenhouses), taken from the article by Toledo and Scnognamiglio (2021), which has the interest of showing all the complexity of the parameterisation of a photovoltaic structure. The ontology is interesting in that the authors separate the spatial organisation of the photovoltaic plant (the repetition patterns of the panels) and the unitary description of the panels (“patch”, the patch corresponds to the single unit of a strip configuring a pattern, i.e. the PV module).
Reason – Spatial arrangement of the photovoltaic tables
o Table size (length, width, area)
o Geometry (parallel strips, checkerboard, islands)
o Type (continuous, dispersed, random)
o Module density (porous, dense…)
Patch – Description of the photovoltaic modules
o Transparency (opaque or semi-transparent/semi-translucent)
o Module size (length, width, surface)
o Orientation (azimuth angle, tilt angle)
o Colour (RAL code, hue, saturation, brightness)
o Border (thick, thin)
o Height from the ground
Energy components of the plant
o Rated power,
o Number of modules,
o Power density,
o Energy intensity of the land use,
o Annual normalised energy production
o PV module technology (single-sided, double-sided)
o PV layer technology (photovoltaic active materials, mono/poly crystalline, spectral selective film
Technical components of the plant
o Type of system (fixed, single/dual axis rotatable),
o Module support system (material, technology, weight)
o Foundation system (material [concrete, wood..], technology, weight).
Agronomic component (the authors speak of the interstitial space between the PV modules and the soil)
o Characteristics of the three-dimensional model or 3D arrangement of plants under the panels (area and volume of planting in relation to the panels, density of plants, ability to move around and under the panels)
o Crop characteristics (type, crop diversity [monoculture, crop combination], plant height, number of months in production)
o Energy characteristics (ground irradiation, yield)
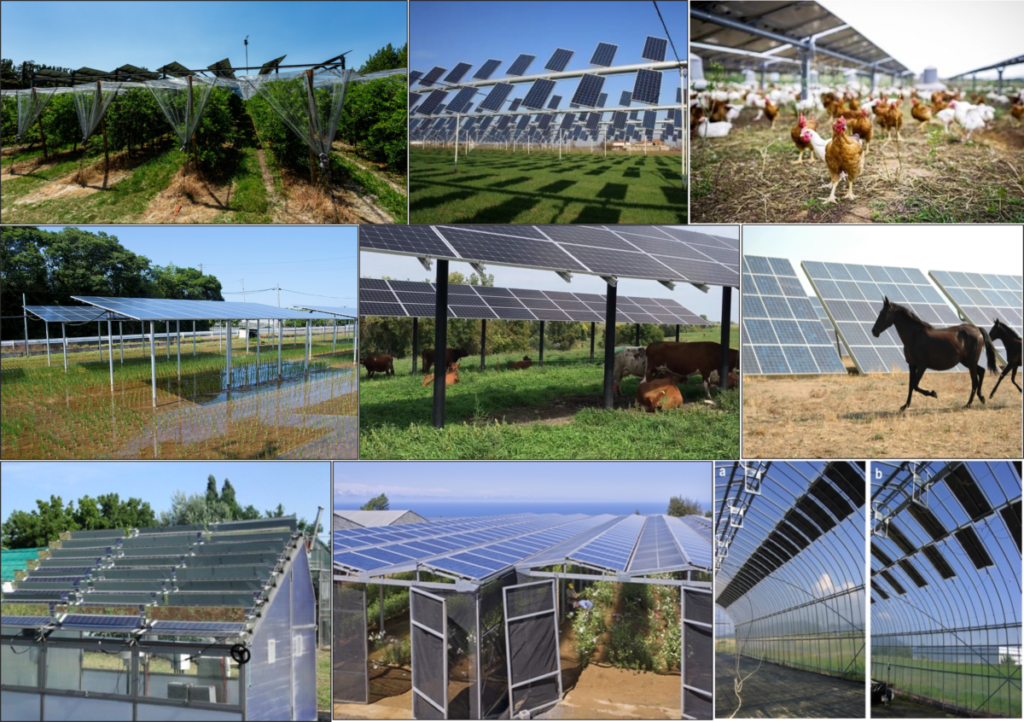
Figure 7. From left to right and from top to bottom: dynamic driven panels with hanging nets, dynamic panels at height, chickens under fixed panels, fixed panels in rice fields, cattle under fixed panels, horses under fixed panels, dynamic panels on greenhouses, multi-hutches, panels installed on existing structures.
Let’s try to take a step back
Different models of agrivoltaism
In reality, there seem to be two very different models of agri-voltaism. In the first model, the main issue is the economic stability of the farm (although there may be irrigation issues). The context is mainly that of extensive agriculture – pastoralism, fodder meadows, cereal meadows, where a high level of technicality may already have been reached. Agri-voltaism is then interesting as a co-activity, but it is necessary to think about something simple, robust, which does not require subsidies, and which makes it possible to maintain the cultural route in place. The agrivoltaism of breeding is an interesting model here, with simple photovoltaic tables that will ensure a spread out growth of grass in spring and summer. This spread will make it possible to limit mechanisation costs in the sense that it will no longer be necessary to stockpile in the spring so that the animals have something to eat in the summer, since the photovoltaic panels could make it possible to ensure a smoother production between the spring (a little less production than normal) and the summer (a little more production than normal because the panels protect against heat waves). This first model is therefore rather low-tech (even if some might disagree with the choice of this term).
The second model is more oriented towards high value-added crops (arboriculture, viticulture, perfume plants, etc.). Here, the main issue is the sustainability of production by maintaining its original qualities (vineyards with varieties that can have the same alcohol content, avoiding or limiting major climatic hazards, etc.). The idea is not so much to maintain the economic activity as to maintain the production with the same level of quality. This model of agri-voltaism is rather technological, costs quite a lot to set up and, in terms of profitability, may require subsidies. In view of the cost of the infrastructure (and the rising cost of raw materials), the yield of the crop under panels must be sufficiently interesting (one can imagine that the buyers of Saint Emilion would not be shocked to pay a few euros more for their bottles anyway). For the prices of these projects to be competitive – compared to a ground-based power plant, for example – it will be necessary in any case to have very large scale effects (because the State subsidies will not be infinite in any case).
This second model could be particularly interesting on land with high agricultural potential to ensure that production is not lost. The economic model is also a bit more refined – we have already mentioned one example with panel control technologies. This dynamic agrivoltaism is seen more as a service. Is it necessary or superfluous? In a dynamic agrivoltaism system, it is necessary to assume a deoptimised electricity production in the service of agriculture. And this model does not have the same economic solidity everywhere. In the north of the Loire, for example, this agrivoltaism model is perhaps not addressable because the penalty is twofold: the sunshine is lower than in the south of France (the panels will produce less energy) and the vegetation will also need the maximum amount of available light (the panels will therefore tend to fade). Shade control is also more difficult to justify in the context of livestock farming. Even with tracker systems in place, the idea is not to optimise shading for the animal, especially as the animal moves.
The two models presented are somewhat at the extreme of each other, and there are obviously a number of models and technologies that gravitate between the two (uncontrolled overhead shade systems, etc.).
The success of an agrivoltaic project requires a change of reference point for the farmer: the light used to produce electricity is no longer used by the plant. The issues to be dealt with are therefore extremely numerous: climatic management, organisation of production calendars, modification of cultivation operations (choice of varieties and densities, fertilisation, etc.), management of marketing channels, organisation of the payroll, financial and legal management, and restructuring of the overall business strategy. And all these issues will be considered differently depending on the location of the farm. The agricultural issues are not the same, the economic stakeholders are not the same, and the sectors are different. In the north of the Hérault region, for example, climate change poses a serious risk to the food security of livestock farmers. Photovoltaic tables can therefore be interesting for gaining several weeks of food security. This context would obviously not have been the same in the north of France or in an area without livestock.
Simplicity and flexibility are perhaps the two key words to keep in mind. Photovoltaic installations are set up for several decades and require farmers to be able to project themselves into the future, to work on questions of transmission and potential changes in management methods. But the integration of a certain flexibility into the system is important because no one has the power of divination. There is no guarantee that the livestock market will not be totally transformed in a decade’s time (mitigation of greenhouse gas emissions in agriculture will, for example, require a reduction in the size of the herd). However, it is still important, even without being clairvoyant, to work on prospective scenarios and to try to anticipate hazards. For example, specific clauses can also be drafted to protect against internal hazards (personal problems, events on the farm) or external hazards (economic, health or sectoral crisis) – whether these difficulties are cyclical or structural for the farm. The provisions must allow the farmer during the duration of the hazard not to be constrained by the project, for example by being temporarily released from some of his commitments until the situation returns to normal.
Should the photovoltaic system be adapted to the agricultural system, or should the agricultural system be adapted to the photovoltaic system? The right answer may actually be somewhere in between…
Are the Multiannual Energy Programmes (MPE) targets unrealistic?
The MPE targets are so far only half achieved (compared to the 2023 targets), so there is no reason to be completely happy. It must be said, however, that the ambitions for photovoltaics are quite high. And to make photovoltaics, you need surface area. So where to find it? There are several types of land that seem rather suitable for this type of infrastructure. For example, there are building roofs, car parks, polluted or unpolluted industrial wastelands (former oil depots, mechanical workshops, former gas plants, tyre storage sites), economic or leisure activity areas where the soil has been stripped, former waste storage facilities and dumps, former mine slag heaps, quarries and gravel pits at the end of their life cycle, former industrial wastelands and military wastelands, or even abandoned areas linked to infrastructures (railways, motorways, airports).
If we add up all these surfaces, we might be tempted to say that the spatial coverage of these lands is sufficient to cover the objectives of the MPE. Let me bring you back to reality. First problem: the majority of these sites are affected by technical or administrative constraints that prevent the implementation of these photovoltaic infrastructure projects. These constraints are mainly due to the proximity of these potential photovoltaic power plants to a historical monument, an airfield, an ecological protection area, or a water catchment area. It is therefore reasonable to expect that impact and environmental studies will be required, which would dampen investors’ interest. Some of these sites, taken independently, are actually quite small and do not allow for the installation of sufficiently interesting power from an economic point of view (because the costs of connection to the grid will have to be added, and the economies of scale are too low). It is understandable that in terms of project management, managing a fragmented asset (with many roofs for example) is more challenging than managing a centralised asset over a large area. The economic aspect can of course also come into play if the sites do not have enough sunlight and if the developers do not consider the return on investment to be sufficiently attractive. It should also be noted that some rooftop sites would not be exploitable because the roofs would not have been designed to support the extra weight of the panels. Some sites also tend to be put aside by developers because they would be too expensive to reinvest or because the environmental issues would be too important (Seveso, PPRT, pyrotechnical pollution). The owners of some sites prefer to convert their sites to urban areas (housing, business parks) rather than install photovoltaic power plants. For others, it is the duration of the installation of the panels on the site that may pose a problem. We can indeed think of commercial activity zones or supermarkets that would not want to install shading on their car park in case they consider extending their commercial zones.
Despite the government incentives in place for these preferential sites (and the dedicated calls for tender from the CRE), it has to be said that they are not enough to ensure both the desired rate of growth and the hoped-for level of competitiveness. Finding land therefore remains a major problem and, insofar as the majority of this previous land has already been prospected, signed or built on, agricultural land remains an interesting alternative for developers – a preponderant share of land in France being agricultural land. Natural areas can be considered – some developers are still prospecting – but one can imagine the complexity of biodiversity issues in these areas. On agricultural land, operators will prefer to set up private contracts with farmers because the calls for innovation from the Commission de régulation de l’Energie (CRE), although financially interesting in terms of electricity buy-back tariffs, limit the surface area of the agricultural projects on which the power plants are located (I refer you to the section dedicated to the CRE calls for tender).
According to estimates, achieving the objectives of the MPE would require the use of between 30,000 and 40,000 hectares of land, all surfaces combined. Currently, ground-based solar power plants represent between 35 and 50% of the installed capacity of the French solar photovoltaic park, i.e. between 5,000 and 7,500 hectares (the other 50% being generally found on roofs). Five hundred (500) hectares would be of agricultural origin. A study by Solagro in 2020 estimates, in very large grids, that between 10 and 50% of this future demand in surface area (to reach the 30,000 to 40,000 hectares required to achieve the objectives of the MPE) will be taken from agricultural land. How should we position ourselves in the face of these figures? Are we dealing with a massive artificialisation of agricultural land due to the production of renewable energy?
The artificialisation of agricultural land is a real problem – and it is all the more topical as power plant projects can compete with agricultural land. There is a fear that some land will be used for other purposes. This competition for land is often at the heart of the debate on renewable energies. Whether for wind power, photovoltaic, biofuels or methanisation, agricultural land has always been seen as a destination point for questions of space and compatibility. And the surface area required for the implementation of these infrastructures is totally linked to the energy density of each of these energies – dense energies will require less space than less dense energies (all other things being equal). Photovoltaics is one of the least dense renewables, but it is also the easiest type of infrastructure to implement. And as we have discussed, France has a fairly strong will – linked to a political context and the use of land without conflicts – to favour roofs and shades or anthropised land (wastelands, quarries…). However, not all agricultural areas are comparable. It is conceivable that in nitrate zones, where the risks of pollution are very high (for example near water catchment areas), the installation of photovoltaic power plants would be less troublesome.
Let us perhaps recall here some orders of magnitude and try to compare comparable things. The French agricultural area (SAU) is around 29 million hectares. Artificial surfaces in France represent nearly 6 million hectares (Terre de Lien, 2022). This includes housing areas, industrial and commercial zones, and all of the country’s road infrastructure (roads, railways, car parks, paths, building sites, wasteland, etc.). It should be remembered that the main factor in soil artificialisation is housing… On average, since the 1980s, nearly 60,000 hectares have been artificialised each year. Looking at these figures, it appears that the potential land area attributed to photovoltaics (a few tens of thousands of hectares) is relatively small compared to the other urbanisation and artificialisation phenomena underway. The idea here is obviously not to defend the photovoltaic power plant projects body and soul, but to share common reading keys and to take a step back on the subject. To go further, would we not even prefer to say that it is much better to have 2% of the useful agricultural area used for real agrivoltaime with all the plots in production under these projects, than to have 0.5% of the agricultural area dedicated to ground-based photovoltaic power plants where all agricultural production has been stopped? Nevertheless, the complexity also lies in the fact that the definition of degraded and artificialized land is not always very clear and may depend on the land use planning document considered.
The 2020 Solagro study also compared this photovoltaic land to the demand for agricultural land to meet the 2007 agrofuel production targets (this is not artificialisation, of course, but it is still energy production). The study shows that ground-based photovoltaics would require 43 times less land than that used for agrofuels in 2007. We should also add that artificialisation does not necessarily mean waterproofing (even if this is often the case overall). In 2014, 2/3 of artificial land was sealed. The question that arises is whether agrivoltaic projects, if they are well constructed, can really be counted as artificialisation, or at least not in their entirety. In addition to the photovoltaic tables, a ground-mounted photovoltaic power plant includes a secure fence, a fire tank, crushed stone tracks for vehicle traffic during construction and maintenance (and potentially tracks for the fire brigade), energy transformation stations, and an energy delivery station. Nevertheless, if well thought out, agri-volta projects should be easily dismantled and the land returned to its original state (even if it is agreed that the original state is, from a theoretical point of view, impossible to return to completely). Generally speaking, the issues of soil sealing are particularly worrying and we can hope that the proposals and measures relating to the law on water will provide some answers. The recent creation of the observatory on soil artificialisation, within the framework of the Biodiversity Plan, is also part of the vigilance mechanisms that will make it possible to continually reexamine these issues and uses.
However, once again, as irradiation is not homogeneous across the territory, it is to be expected that some agricultural land will be much more densely populated than others. For example, full power irradiation would be around 2000h for Reunion, 1000h for Paris and 1500h for the South East of France. Let us also add that many agricultural lands are, by their nature, out of interest for the main developers: agricultural lands with a too strong slope or lands where the slope is oriented North. Further refinement could be made by determining the types of agricultural areas that are relevant for agri-voltaics: perennial crops under climatic stress in the south of France, permanent grasslands to improve animal welfare and feeding,
Towards agri-voltaic projects anchored in the territories
The objectives of the MPE must be implemented at the territorial level, where agri-voltaic projects must be properly integrated. There are many regional planning documents: urban planning (SCoT, PLUi – French acronyms), energy and climate planning (PCAET, SRADDET – French acronyms). And the territories are putting forward strategies (admittedly sometimes totally unrealistic) of positive energy regions and territories (acronym TEPOS – French acronyms) or carbon neutrality. It is clear that the subject of renewable energies – and in particular photovoltaic energy – is thus widely addressed in these planning documents. And when it is combined with the objectives of carbon storage in agricultural soils, one is rarely disappointed… (interested readers can find a blog post on this subject about carbon in the soil) Some people may need to be reminded that just because one sends electrical energy of renewable origin to the network thanks to installed photovoltaic panels does not necessarily mean that one is consuming renewable electricity (one does not choose the electricity of one’s network).
The integration of the agrivoltaic site in a territorial project is important and it can be materialized in many different ways. For example, it will be possible to have a CUMA (Cooperative for the Use of Agricultural Equipment) work locally or to support it so that it can, for example, build a shed, develop a local industry or outlet, or even think about setting up a local slaughterhouse if an agrivoltariat project is launched. The income from agrivoltaism could also be used to finance a contribution to the actors of the agricultural sector of the territory to allow a direct support to the local fabric, a help to young farmers in the creation or the resumption of exploitation, and more generally a greater acceptability of the project by the inhabitants of the territory (citizens, economic actors, elected representatives…).
Photovoltaics must not be a disguised subsidy for the farmer. Some malicious photovoltaic operators will tend to give priority to farmers who are suffering to ensure a faster acceptance of photovoltaic projects. It is perhaps these projects that have the greatest risk of being alibi projects. The farmer (or group of farmers) must be a strong stakeholder in the agri-voltaic project and should even be at the origin of the project. By investing financially in the project – i.e. by also taking their share of the risk – farmers will have every interest in having a serious agricultural project in mind. And the grouping of farmers within the same project is extremely relevant. By sharing land, a farmer’s collective will, for example, have the capacity to launch a project that each farmer could never have launched independently.
Several surveys suggest that the population would be rather favourable to the implementation of photovoltaic parks on agricultural land, as long as they are really considered as agri-voltaism. However, one of the black spots for the population is the landscape impact of such plants. The integration into the landscape remains the most difficult to manage. With its several meters high fences and its sometimes impressive photovoltaic tables, photovoltaic power plants can be frightening, and sometimes give more the impression of an industrial site than anything else. The French are very attached to the landscapes and charms of their territory (and to their panoramic view from their houses, villas and swimming pools). Will they be prepared to take the fall? Some regions – think of the AOP (protected designation of origin) and AOC (appellation of origin) vineyards, for example – have staked everything on the landscape and tourism. It is difficult to imagine power plants installed on several hectares of vineyards without strong confrontation with local stakeholders, even if these power plants are supposed to protect the vines from climatic hazards… Add to this the fact that, given the distribution of irradiation over the French territory, photovoltaic power plant projects will tend to be unevenly distributed over the territory, which will not necessarily help in terms of local confrontations. With procedures for the creation of power plants lasting several years, one must be patient. Some farmers will even have had the pleasure of receiving some sharp criticism regarding the implementation of integrated and well-constructed agrivoltaism projects on their farms, which, according to local residents who are obviously experts, would limit biodiversity.
In some national parks, some photovoltaic projects have taken the lead and now offer educational tours and information panels around the plants to explain to passers-by what they are all about. Some developers are also considering removing the fences around their plants, especially on projects where the PV panels are high up (it should also be said that once the panels are already mounted and installed, they are harder to steal). More and more project owners of all sizes are also calling on participatory and citizen investment to share in the profitability of agricultural projects, with the underlying idea of improving the quality, transparency and acceptability of these projects. These investments remain, however, in the vast majority of cases rather low in relation to the total price of an agrivoltaic power plant.
More generally, photovoltaic power plants show what the population seems to have forgotten. Energy production is not immaterial, and it can take up space. Local residents are generally in favour of producing energy – if it is green – but not too close to their homes.
Normative and regulatory approaches not yet stabilised
Between doctrinaire vision and laissez-faire
Agri-voltaism does not leave everyone indifferent, to say the least. We are currently seeing an increase in the number of local charters on a wide variety of territorial scales, with some charters having overlapping perimeters. Some local authorities have drawn up charters in connection with their planning or development documents (Scor, Plu, PAT, etc.). Others have been proposed by the main agricultural unions (FNSEA, ACPA) and by sector unions (FNO, Synalaf, etc.). A national doctrine is also being developed.
Many state and para-public structures tend to slow down the deployment of the agri-voltaic sector under the cover of a sometimes rather doctrinaire and ideological vision. Some departments, overwhelmed and submerged by requests, prefer to keep a very narrow framework of acceptance of agri-voltaic projects to cope. Other authorities (chambers, DDT…) are afraid of finding themselves with hectares of photovoltaic projects on their territory and of being held responsible for them. But can we really blame them? The bad experiences of the past have left a bitter taste in their mouths. The actors of the territory keep in mind the French photovoltaic greenhouses, well financed but very badly conceived for the development of an agricultural production underneath. And the German examples, where methanisation replaced livestock farming (cows were replaced by maize for methanisation plants following particularly attractive purchase contracts) will also have been widely talked about.
In France, until 2011, all photovoltaic projects, whether on rooftops, ground-mounted parks or shaded areas, benefited from a guaranteed purchase rate for the electricity produced, set by the State for a period of 20 years. This means that some clever people have taken advantage of this to make a nice little sum of money. The image of the sector was largely tarnished when numerous photovoltaic greenhouses, built by electricity producers, forced many market gardeners to stop their agricultural activities and file for bankruptcy. At the time, the photovoltaic tool was primarily designed to produce electricity and the agricultural component was not at all on the agenda. Some farmers also invested in numerous greenhouses by creating multiple photovoltaic project companies without really setting up any agricultural activities underneath. These greenhouses, largely obscured by light and actually looking more like storage sheds than agricultural production areas, were then used mainly for energy production and are now completely abandoned. It is estimated that between 60 and 80% of agricultural photovoltaic greenhouses are no longer used for agriculture (and these projects have not been sanctioned). These projects are now prohibited. Similarly, some actors will have seen these guaranteed feed-in tariffs as a great opportunity to create buildings (agricultural or not, oversized or empty) compatible with the open window (less than 100 kW) simply to install photovoltaic panels on their roofs. In 2011, following a report by the Cour des Comptes, the State put in place a moratorium on photovoltaics to stem the speculative bubble and introduced a new framework for supporting projects, which is revised regularly (I refer you to the section on CRE calls for tender at the beginning of the dossier). The blow dealt by the State is brutal and many companies that had launched photovoltaic projects and hired employees are forced to close their doors
Some agricultural authorities are also wary of the arrival of major electricity producers and large market developers. As agri-voltaic projects are not eligible for CRE calls for tender – except those dedicated to innovation – photovoltaic developers tend to be interested in large agricultural areas. In particular, ground-mounted projects covering several hundred hectares have recently been launched. For photovoltaic developers, negotiating by mutual agreement with farmers, outside of CRE tenders, is much easier to manage than public tenders for degraded land. In particular, there is less competition and the costs of preparing the agricultural land are lower than in the case of former extraction or landfill sites, which require major levelling and decontamination work or specific anchoring.
The arrival of these electricity producers is also motivated by the objectives of the multi-annual energy programming (PPE) but also by all the industrialists in the economic sector who are asking to have part of their energy consumption covered by over-the-counter contracts (PPA). These industrialists know that they will have to reduce their carbon footprint anyway, at the risk of significant penalties, and are willing to source their energy from renewable sources.
Many players – energy companies or not – are involved in agrivoltaism for opportunistic reasons, seeing behind these projects the capacity to be deployed on agricultural land. Between greenwashing and alibi production, we must not be taken in. Some do not know the difference between a landowner and a farmer, others plan to organise transhumance of sheep under a panel, and still others do not consider the issues involved in handing down farms to the new generation of farmers. Not all operators are receptive because the constraints of agrivoltaic projects can be important (number of panels, spacing between panels, different types of technologies adapted to agricultural production routes, etc.). Large electricity producers are not always the most coherent either, as they might tend to see this subject of agrivoltaism on a very large territorial scale. We have seen that it is difficult to generalise the results of agri-voltaria projects. Nevertheless, remaining objective, one could add that several of the large producers who have embarked on renewable energy production have a long tradition and historical presence in France. Some of them have been able to develop an understanding of the French agricultural environment and have become aware of the complexity of the development of such projects (operational and logistical constraints of the farmer on the spacing and size of the panels, the farmer’s self-consumption strategy, taking into account the farmer’s cultivation itinerary, etc.), with the desire to build projects within a framework that is specific to and validated by the territories.
At the risk of remaining in a rather reductive vision of agrivoltaism and looking only at the bad experiences of the past, it could be a pity to let well constructed agrivoltaic projects pass by. Whether it is on the implantation of power plants on land that is anyway not very fertile and productive or on projects shared by several farmers generating new agricultural jobs and well established on the territory, the possibilities are numerous. The visions and doctrines on the territory remain quite different. In any case, the bodies are quite heterogeneous on the territory (in terms of unionism, open-mindedness, chauvinism, etc.). For example, some chambers strongly support the development of agri-voltaism. In the end, this may not be too much of a coincidence, especially in the centre of France (e.g. in the Limousin, centre of France) where suckler production barely provides a living for farmers and raises questions about the sustainability of breeding, the lack of alternatives to suckler farming, and the renewal of the farming generation. For some, it is better to set up agrivoltaic projects than to be in regression on livestock farming. In view of the low remuneration of farmers, one can hardly blame them for wanting to supplement their income by using part of their farmland for agri-voltaic projects, as the rental income can be much more attractive than the agricultural production itself. However, one may wonder whether the problem is not much broader than that: dominant agricultural models, low remuneration of farmers, long supply chains, etc.
Let us nevertheless agree on one thing: alibis and patrimonial files must be blocked. Some authorities are also inundated with files involving communal land, as some elected officials have seen this as a financial windfall for their territory, without necessarily involving the farmers…
A lack of regulatory framework
At present, it seems relatively difficult to retrieve geographical information on the location of photovoltaic projects by institutional actors, nor to know the exact number of finalised projects (Acte Agri Plus would have identified more than 200 in Europe in 2021). This difficulty is mainly due to the fact that agri-voltaics is not defined by law or regulation. The creation of an observatory for agri-voltaics, proposed by the National Assembly during its flash mission, should help clarify matters.
Without a regulatory framework, the interpretation of the law remains open to anyone and everyone involved, and opportunists are never far away to get their alibi projects accepted. One may worry about the rather loose definitions of agrivoltaism. Once again, no one seems to be doing much of anything. No guide (whether from ADEME, AFNOR or others) is currently retained as the reference guide in which one could find the indicators that rule on the viability of an agrivoltaic project in its agricultural dimension. This is understandable, as the stakes are so high for the players in the sector. It must be said that lobbying is quite powerful between the SER, France Agrivoltaisme and Solar Europe, which include many electricity producers. But the public decision-makers must take the subject in hand and make the decisions objective. A national charter on the subject is said to be underway. Decision-makers will also have to trust local actors who will be able to assess the quality of agri-voltaic projects on a case-by-case basis. Other countries in the world have been able to agree a little more (e.g. Japan), by proposing for example that agricultural production should not fall below a threshold of 80% in relation to an initial reference (it is still necessary to be clear about this reference), by fixing a minimum height of the panels so that agricultural machinery can pass underneath them, and by allocating temporary permits for the conversion of agricultural land.
The regulatory framework is certainly necessary in order to limit abuses (we do not want to see mega-projects that would favour certain types of agriculture), but it must not be a brake on the development of agri-voltaic projects. We could also add that the objectives of the MPE are still far from being reached and that the pace of administration of the files does not make the task any easier. Launching a photovoltaic project takes some time and we have seen that the urban planning, energy and environmental codes are sometimes contradictory. Several proposals have been made to simplify the administrative process around agri-voltaic projects by promoting the harmonisation of procedures and decisions between different territories, the sharing of experiences of local actors, the accessibility of CRE calls for tenders to less innovative projects, and by limiting the constraints to the deployment of photovoltaic projects such as the need to submit building permits when the photovoltaic project is not too important. It will nevertheless be important to ensure control on the ground – we have seen that local structures (notably the CDPENAF) could play this role. And many criteria could be considered, either to favour the most virtuous agri-voltaic projects in calls for tender (with, for example, agronomic bonuses, just as there are bonuses when projects are carried out on degraded land), or to make aid, additional remuneration or compensation conditional on projects that are solid and anchored in their territory: agronomic criteria, criteria linked to the land area, criteria linked to agricultural development objectives in the regions, or criteria linked to the projects’ business model.
Feedback from experience still too weak
Unlike the agricultural world, the energy industry is not necessarily used to experimenting. Experiments do take place with certain technologies, within the framework of certain co-activities, but not always with a scientific methodology that is not that of the developer.
Currently, very few photovoltaic parks are really adapted to agrivoltaism. It is therefore difficult to create data and knowledge. In view of the little data currently available, confidentiality issues are high. Those who have some data are not really inclined to share it, firstly because they do not necessarily know what they are giving (they do not have long-term data) and secondly because they know even less what they could recover (if other operators provided them with their data) and how the information will be disseminated. Some countries, such as Israel, have reportedly opened calls for tender with categories dedicated to agrivoltaism for many small-scale projects; a way for them to multiply projects on the territories and thus collect a lot of data. This lack of data may seem rather paradoxical when we see that some experimental projects are equipped with sensors. Do we really need to deploy so many detailed measurements? Do we really need cameras to monitor the growth of the grass under the panels?
There is general agreement to recover knowledge, but when it comes to investing money to generate that knowledge, it is a different matter. Some producers will agree to carry out relatively simple monitoring but will find it difficult to integrate these costs into their business plans. With the steering and monitoring committees of new agri-voltaic projects – committees requested by chambers of agriculture or by operators – it is as much time to wait before being able to have truly exploitable results.
With the diversity of agricultural projects, it is relatively difficult to generalise results. The question is whether, given the multitude of farms and cropping patterns that exist, the stakeholders will have enough data to make serious statistical analyses after a few years. Soil and climate conditions, cattle breeds, crop rotation and cropping patterns are all variable parameters that are not necessarily standardised. As we have discussed extensively in this dossier, it is difficult to be entirely confident about the impact of panels on biomass production, the selection of the most suitable species for photovoltaic installations, the impact of the plants on animal welfare or the most optimised technological devices for a given production (width and inclination of the panels, height of the structures, etc.). For the time being, perhaps we should rather adopt a position of humility and accept that we don’t know everything.
Is it really that easy to launch an agrivoltaic project?
Let’s perhaps also add the specificity of France with regard to its agriculture (we are a country that is very proud of its land) and solar energy (with a rather preponderant nuclear imagination), two parameters that distinguish France quite strongly and that foreign energy companies and developers do not necessarily understand when they come to the country. The French specificity, with the devices of the energy regulation commission, and the administrative mechanisms deployed at the local level (CDPENAF, local authorities, Chambers…) also protect France from foreign or speculative players who would come to the French market and push for a uniformity of the agrivoltaic market.
Most photovoltaic power plant projects take between 5 and 10 years to see the light of day, which means that there is no risk of seeing a power plant spring up on a plot of land in just a few moments. For the time being, it must be said that there are relatively few rules and, when there are, they are not necessarily complete or harmonised. From a regulatory point of view, the installation of photovoltaic installations must juggle between the energy code, the town planning code and the environment code. The obstacle course begins at the administrative level.
We have already talked about the energy code, with the calls for tender to the CRE and the private contracts on the markets. This code roughly defines how to connect to the grid and at what price the photovoltaic operator will have his electricity bought back (this is also when we made the difference between compulsory buybacks, agricultural compensation, and supplementary remuneration for operators…).
Photovoltaic installations on agricultural land are regulated by the town planning code but the provisions of the town planning code are less restrictive and detailed than those of the CRE. Agricultural land is basically not suitable for building. However, the town planning regulations can be adapted under certain conditions so that the land can be built on, in particular by adapting communal maps and local town planning schemes (PLU or PLUi). In agricultural (Zone A) and natural (Zone N) areas of the local urban plans (PLU), only constructions and installations necessary for farming (and forestry in Zone N), public facilities or public services (CINASPIC) ‘as long as they are not incompatible with the exercise of an agricultural, pastoral or forestry activity on the land on which they are located, and that they do not affect the preservation of natural areas and landscapes’ are authorised. In other words, the fields of interpretation are quite open for requests for urban planning permission. It is only a question of demonstrating the compatibility of the photovoltaic equipment with the exercise of an agricultural activity (or the need for agricultural production), without dealing with its impact on the quality of the latter.
Do you want more? No problem. We can also add considerations around the “Mountain” and “Coastal” laws in the case of municipalities bordering the sea or the mountains, which could block the installation of photovoltaic power plants, the latter being considered as extensions of urbanisation and having to be implanted in continuity with the built-up area (and therefore not necessarily close to an agricultural parcel). Without a specific derogation – wind turbines, for example, have a derogation in these cases – it is impossible to install photovoltaic power plant projects in an agricultural context in the municipalities subject to these laws
The complexity of the link with urban planning documents also lies in the typology of photovoltaic installation projects, because not all are on the same level:
- Ground-mounted solar power plants with a peak power of less than 3 kW and a height of up to 1.80 m do not require a building permit
- Ground-mounted photovoltaic power plants with a peak power of less than 3kW and a height of more than 1.80 m and those with a peak power of between 3 and 250 kW are subject to a prior declaration.
- Other photovoltaic projects with a peak power of more than 250 kW have to prove the compatibility of their project with the agricultural component to the State’s instructive services and are obliged to apply for a building permit and to carry out an environmental assessment.
By judging the compatibility of a photovoltaic project with the agricultural component, the prefectures, the regions, the CDPENAF (commission for the preservation of natural, agricultural and forestry areas) and the municipalities can thus accelerate or slow down the deployment of photovoltaic installations at local level. The CDPENAF – which we regularly hear about in relation to agrivoltaism projects – has in fact more of an advisory role here. This body issues an opinion on any urbanisation project that could affect natural, forestry and agricultural areas and on the means to contribute to limiting the consumption of these areas. Through their local presence in the territory, the CDPENAF has the capacity to assess the quality of agrivoltaic projects on a case-by-case basis, and to give an opinion on the relevance of such projects on the territories. Some actors even tend to push for an increase in the powers of the CDPENAF (for example by giving them the competence to issue Certificates of Eligibility of the Site on Agricultural Land for CRE tenders, which would currently be done by the DREAL; or by requiring projects that are not subject to an impact study to write an assessment note that the CDPENAF could analyse).
Building permits can be state permits (signed by the prefect) or municipal permits (signed by the mayor). At present, the vast majority of permits granted are state permits, although Barbara Pompili – former Minister for Ecological Transition – had declared that municipal permits would be opened up more widely. However, the fact that some municipalities seem to be completely overwhelmed by events raises questions about the use of municipal permits. Once the building permit has been submitted, the story is still far from over. This long period of time nevertheless allows time to prepare, to build up the mayonnaise, and to set up a coherent project, and as a group. Nevertheless, it would seem that whatever the state’s instructive services say, good lawyers could get a refused building permit accepted. According to some interpretations, a photovoltaic power plant could be considered of public utility as a response to the objectives of the energy transition, thus sweeping away potential refusals from municipalities and prefects.
Land management also brings to mind the SAFER. The SAFERs would rather intervene upstream to make sure that ill-intentioned actors do not come and buy the land. However, when there is no land purchase, agri-voltaic projects are rather organised around long leases (the land then remains in the hands of the farming community), and the SAFERs have little direct input. The actors of the territory would rather tend to fight so that the agrivoltaic projects remain on agricultural zones in the urban planning documents. By losing the agricultural notion of the land, there is a great risk that at the end of the agri-voltaic project, the agricultural land will not be returned to agriculture (this is what many agricultural services fear). One could also add that, not knowing the energy available in the coming decades, it is important that the land remains in agricultural hands. Nevertheless, it is reassuring to know that in France, agricultural land is still fairly protected (the situation is quite similar in Italy, which has very strong ambitions for the development of photovoltaic energy and a fairly restrictive vision of the use of its land).
For power plant projects with a sufficiently large capacity, an environmental assessment is required – particularly with regard to the impact on local flora and fauna. These impact or environmental studies, which can be complemented with pre-feasibility and landscape studies, are sometimes criticised at the time of submission of the project to public enquiry. Since 2014 and the Loi d’Avenir pour l’Agriculture (Future Law for Agriculture), the legislators have also imposed the implementation of preliminary agricultural studies (EPA), often carried out by chambers of agriculture or private consultancies. These EPAs are a kind of agricultural audit which aim to provide information on the interest of the photovoltaic project from an agricultural point of view, to present the measures envisaged to avoid and reduce the negative impacts of the installation, and if necessary, to define the collective compensation measures aimed at supporting the agricultural economy of the territory. This audit is an opportunity to consider the agricultural territory (opportunities from an agricultural point of view, outlets and sectors, traditional practices, appellations), to analyse the farm (production, workshops compatible with agri-voltaism, needs, strengths, weaknesses), to evaluate if the project is well designed for the farm and if it allows to answer potential weaknesses of the farm, and finally to evaluate if the agri-voltaic project fits well with the territory and its aspirations, and if the project can answer potential problems of the farm.
France is also committed to achieving the goal of Zero Net Artificialisation (ZAN) by 2050. Under the guise of combating soil artificialisation and the loss of agricultural production potential, regional planning documents, particularly the Schéma de Cohérence Territorial (SCoT), can slow down the development of photovoltaic systems in agricultural areas. And to add that the expression “consumption of space” is not explicitly defined in the town planning code. It is therefore up to the authors of these documents to define the methods for calculating the consumption of natural, agricultural and forest areas, which may lead them to include photovoltaic panels. A provision of the climate law would stipulate that a natural or agricultural area occupied by a photovoltaic energy production facility is not counted in the consumption of natural, agricultural and forest areas. This is enough to make you goat…
In short, in the vast majority of cases, setting up agri-voltaic projects is no easy task. There is even a decree limiting to fifteen consecutive days the period during which a non-agricultural activity can be carried out on a plot of land benefiting from the CAP. Clearly, this decree is in complete contradiction with an agrivoltaism project. Some people would even take advantage of this administrative complexity and the money from the photovoltaic sector to sell studies – it has to be said that between impact studies, preliminary agricultural studies and technical and economic studies, there is a bit of money to be made (we have also seen archaeological excavation projects and the intervention of actors around historical monuments)
From agrivoltaism to ecovoltaism
The impact of agrivoltaic power plant projects on biodiversity is still relatively unknown. Few robust lessons can be drawn from agrivoltaic projects, especially since the initial situation (reference situation) is not easy to take into account. Some work will have revealed a decrease in species richness and a change in the floristic composition of the canopy under the PV panels. Nevertheless, these biodiversity considerations are not completely neglected. And these projects would not necessarily have a negative impact – beware of jumping to conclusions. Several developers and/or experimenters are setting up monitoring systems and dedicated infrastructures: construction of habitats, creation of ponds, biodiversity monitoring, deployment of ecological corridors, installation of nesting boxes for bats and titmice. Power plant projects are also an opportunity for some farmers to make the transition to organic farming and other more virtuous practices. Beware, however, of small-scale power plant projects that are not subject to impact studies… Environmental associations are said to be in the process of creating reports – France Nature Environnement (FNE), La Ligue de Protection des Oiseaux (LPO) among others.
The concept of ecovoltaism has even been recently proposed. Behind this term is the development of unused surfaces under solar panels (particularly on low-lying power plants with grassland production) in a programme of applied ecological science. These unused areas could be used to promote the restoration of endemic plant communities and the installation of plants intended for biodynamic agriculture (eagle fern, borage, rhubarb, borage, comfrey, etc.), melliferous plants (sweet woodruff, bellflower, broom, lamier, lemon balm, hyssop, etc.), endangered plants (classified in the red list by the IUCN) or plants of medicinal interest. These plants, which must be particularly well selected – for their resistance to shade, the good nutritional quality of their pollen and nectar, or their extended flowering period – would be interesting to plant in difficult growing conditions, with poor and unfertile soils, where the solar panels are fixed, non-orientable and very low, and for which the sites of implantation are subject to climatic hazards (droughts, exposure to the wind, etc.) Although these projects seem theoretically very promising, one could nevertheless question their logistics and their operationality by farmers and by photovoltaic developers.
Between recycling and life cycle analysis
Despite all the rhetoric about the life cycle analysis of photovoltaic power plants, there are no real examples of dismantling that have been carried out. The power plant infrastructure must be completely dismantled and project developers are required to restore the soil to its original state; the costs of dismantling must normally be provided for at the start of the project and covered by the energy company (and therefore integrated directly into the energy company’s business plan). It should therefore not be possible to concret, nor to rake – even if some relief is proposed for concrete to anchor the piles in the ground (some anchors need to be strong for high table structures, there is not always the depth to drive a pile into the ground and some places are in any case more favourable than others to drive a pile). Nevertheless, we have seen some developers take surfaces, completely levelling the ground and leaving it in a destroyed state. This principle of reversibility is a safeguard to prevent the agrivoltaic project from being a first step towards a subsequent agricultural abandonment by hindrance (residues of the installation hindering the exploitation, degrading the production or threatening the safety of the farmer).
Recycling at the end of the plant’s life will become a major issue (the Soren association is in charge of this collection and recycling, with 2 plants in France for Europe). Module and inverter manufacturers are obliged to collect and recycle their products. Theoretically, up to 95% of the mass of a photovoltaic module could be recovered. However, we should not be too hasty. We are talking here about the percentage of potentially recoverable mass and not the percentage of recoverable elements (silicon in particular is difficult to recover). Let us also add that recovery is not necessarily recycling… At present, it is not possible to manufacture a new photovoltaic panel from a photovoltaic panel at the end of its life. Although the theoretical share of recovery of end-of-life panels is generally very high, there are not yet sufficient initiatives – and a sufficiently structured market – to amortise the cost of installing a recycling plant. Some panels would even have a longer lifespan than initially expected – sometimes more than 30 years instead of the 20 years announced – thus extending the quantity of panels for potential recycling.
The environmental balance of agrivoltaism can be considered in two distinct frameworks, the first where all the energy is sold to the grid, and the second where part of the energy produced is (self)consumed on the farm. Some operators and consultancies are even beginning to use the argument of photovoltaic tables in agriculture in a carbon balance integrated in the farms in the sense that photovoltaic installations would allow to change the energy mix on the farm by limiting the use of fossil fuels. It is also possible to make the installation of photovoltaic panels conditional on changes in agricultural practices towards carbon-storing practices (by reducing the risks or the mental burden for the farmer thanks to the long-term investment permitted by the panels). From an accounting point of view, these avoided and/or stored carbon emissions could be partly attributed to the photovoltaic infrastructures.
For information, the Electricity Map website allows a series of indicators on a country’s electricity mix to be viewed in real time. However, this tool does not allow for an assessment of the average carbon footprint of electricity. Indeed, depending on the time of day and the time of year, the instantaneous footprint can vary significantly, particularly when gas and coal power stations are called upon to meet peak consumption. In practice, renewables do not substitute for an average electricity mix, but rather for the last energies called on in an “order of merit”. Not all energies have the same injection priority, renewables are called first, followed by nuclear, then gas and coal. To assess whether photovoltaics can reduce the average carbon footprint of electricity, we prefer to simulate hour by hour what the French electricity mix would have been without photovoltaics.
Let us also add that, if we place ourselves in the framework of a complete life cycle analysis, work must be put in place to reduce the toxicity of the metallic elements used in the photovoltaic tables. The technology of some second generation CIGS (copper, indium, gallium, selenium) photovoltaic cells involves elements such as cadmium sulphide. As cadmium is a highly toxic metallic element, research is underway to replace it with other elements (Zn, Mg, O, S…)
Grid connection and electrical stability
One of the main limiting factors of agrivoltaism for project developers is the distance of connection of photovoltaic power plant projects to the existing electricity grid. Self-consumption and local use of the energy produced is indeed quite rare (except when there are transformation tools next door or, indirectly, to recover the heat produced by the photovoltaic panels to improve the efficiency of a heat pump in an agricultural building), contrary to what could be found in the context of micro-methanisation, and the energy produced will rather tend to be put back into the grid.
The electron goes as short as possible into the grid. This physical constraint implies that the grid connection distance cannot be neglected. As the energy cannot be fed back into local low voltage areas, it is necessary to find a substation to feed the energy back into the circuit. Sometimes it is necessary to dig trenches several kilometres long to reach these feeder stations, and this is not always easy for investors. And for good reason: it costs about €100,000 per kilometre of connection! And the further away the connection point is from the power plant, the larger the power plant will have to be to compensate for the investment cost (a lower density of photovoltaic panels means more cabling in proportion). And the larger it is, the more likely it is that the plant will be a major threat to the sustainability of the farm’s main activity. And to add that in rural areas, the connection points are most often at the end of the network
Moreover, some connection points do not have the capacity to accommodate additional mega watts. The website “capareseau” (https://www.capareseau.fr/) shows the capacities for the connection of electricity production facilities to the transmission and distribution networks. Although a certain amount of the energy connected to these substations must come from a renewable energy installation (under the Schéma Régional de Raccordement au Réseau des Énergies Renouvelables -S3REnR), the voltage zones will most certainly be close to these source substations. Can we raise the voltage of these source stations to accommodate more projects? Aren’t the lines already saturated? These are all questions that can reasonably be asked…
Let’s not neglect the issue of the stability of the electricity network – the balance network (at the beginning of 2022, the electricity transport network RTE warned about the balance between electricity consumption and production following a drop in temperatures). The current networks were designed to have centralised energy production systems. If we imagine several thousand agri-voltaic power plants in place, a lot of work will have to be done on the grid infrastructure to accept new plants and move from centralised to decentralised. Would this completely destabilise the grid? It would seem that there is a scientific consensus on the existence of technological solutions to maintain the stability of the electricity system. These technological solutions would not be necessary today because they would need to intervene if intermittent energies such as solar represented, in terms of instantaneous production, more than 60% of total energy production (we have seen that this is still far from being the case). In any case, there will only be one grid and everyone will have to be connected to it. If everyone did their own private network, it is reasonable to imagine that the states would be creative in proposing dedicated taxation systems.
If one imagines an electrification of agricultural work (with machinery running on electric power) and that one needs to bring electricity to the plot, it could be envisaged to mutualise the networks. By passing a first line from an agrivoltaic power plant to reinject it into the existing network, another line could be passed in the other direction to supply, for example, an electric recharging station on the plot. Microgrids could thus be seen as a stimulus both for local development in rural areas and for eliminating the need for new power lines.
Are we resilient enough?
The use of solar panels at the heart of agrivoltaism projects questions our dependence on solar panel suppliers. Our gaze immediately turns to China, which alone accounts for the top 10 suppliers of photovoltaic panels worldwide. Initiatives are underway in Europe (Holland, Germany, Spain…), but the production capacities are not comparable by far…. The debate on dependence is much wider than just at the French level, and this dependence can only be reduced when states decide to make large capacity plants economically viable. Photovoltaic operators will be able to turn to European suppliers if their photovoltaic projects are sufficiently profitable (and if the panel renewal rate – sometimes more interesting for Europeans than for the Chinese whose energy mix is very carbon intensive – to limit their carbon debt). Sometimes it is also simply logistical issues (such as the availability of panels) that will reinforce the choice of photovoltaic operators towards suppliers.
In addition to this very specific dependence, it is our dependence on energy that is important to consider, particularly that of the agricultural sector. In France, almost 45% of final energy comes from oil (28%) and gas (15%). Agriculture, as it is carried out, is completely dependent on oil energy (Harchaoui & Chatzimpiros, 2018). This dependence does not protect the agricultural population against fuel price increases – a risk now recognised in view of the largely passed peak oil. The current Russian-Ukrainian conflict is also the cause of an impressive increase in the price of fertilisers (produced from gas) – thefts of fertilisers have reportedly been recorded in France… The complete electrification of agriculture is far from obvious (due to the nature of current agri-equipment and the energy demand that the production of fertilisers with renewable energies would represent), but the development of renewable energies will make it possible to respond to some of these dependency issues. The need for sobriety in the agricultural sector – whether through a complete change of model, complete changes in agricultural itineraries or lighter agri-equipment – is obviously very important, but this subject is beyond the scope of this blog entry.
If we continue to take a step back, we could be concerned that our energy system is not resilient to the climate change we are experiencing. Already, without mentioning photovoltaic power plants, the increase in temperature can reduce the efficiency and maximum capacity of thermal power plants. In addition, spatial and temporal variations in rainfall (and rainfall levels, of course), and the temperature of water resources can limit the operations of hydroelectric and thermal power plants. On the photovoltaic side, the outlook is not necessarily good either. The increase in air temperature affects the production potential of the panels. The temperature coefficient of the panels, i.e. the rate of decrease in the performance of the panels for each degree of temperature, would decrease by about 0.3 to 0.5% for each additional degree of temperature above 25°C. With the expected climate projections and the recent IPCC reports of 2021 and 2022, the long-term efficiency of agrivoltaic installations in terms of energy production might be overestimated. However, it could be argued that if the projects are well constructed, these panels should continue to protect crops from increasing climatic hazards, even if their energy production is reduced.
By way of conclusion
By combining crop production and electricity production on the same agricultural area, agrivoltaism is a new practice of cohabitation and agricultural synergy. The agri-voltaic sector is still in its infancy and still lacks clear normative and regulatory approaches. The lack of a clear definition and framework is quite glaring. Although the issues of land artificialisation, agricultural abandonment and land grabbing are real, the fact remains that agrivoltatism cannot be seen from an overly simplistic angle. These schemes are also a way for farmers to invest or finance agricultural tools, to perpetuate a farm, to secure land, or to provide a form of diversification where there are relatively few alternatives. It will be important to ensure that in crop production, the new agrivoltaic projects do not suffer from all the setbacks that the sector experienced with the first photovoltaic greenhouses, and that in animal conditions, these projects are not used for renting equipment with legs. Grazing has never been an agricultural activity in itself.
Agri-voltaic projects should be eligible for all sectors, whether for animal or crop production – even for those that have not yet converged to a relevant agri-voltaism model.
Soutenez Agriculture et numérique – Blog Aspexit sur TipeeeBibliography complementary to the interviews
Acte Agri Plus (2022). Recensement 2022 des projets agrivoltaïsme au niveau mondial : https://aa-plus.fr/agrivoltaisme-2
ADEME (2021). Caractériser les projets photovoltaïques sur terrains agricoles et l’agrivoltaisme. Etat de l’art bibliographique
ADEME (2021). Caractériser les projets photovoltaïques sur terrains agricoles et l’agrivoltaisme. Guide de classification des projets et définition de l’agrivoltaisme
ADEME (2021). Caractériser les projets photovoltaïques sur terrains agricoles et l’agrivoltaisme. Recueil de retours d’expériences et fiches techniques récapitulatives
Afnor (2021). Label Projet Agrivoltaique. Référentiel de labélisation des projets de classe A sur culture. Version 1.1 – Décembre 2021
Assemblée nationale (2022). Mission d’information Flash sur l’Agrivoltaisme.
A’Urba (2022). Photovoltaïque au sol en Nouvelle Aquitaine. Quelle utilisation des sites dégradés et artificialisés ? Quelle acceptabilité des installations en milieu agricole ?
CNRS (2022). Le solaire photovoltaïque en France : réalité, potentiel et défis. Des questions et des réponses préparées par des chercheurs et des chercheuses du CNRS et de la Fédération de recherche du Photovoltaïque.
ENCIS Environnement (2021). Analyse de la concurrence entre les parcs photovoltaïques au sol et les autres usages des sols. Focus sur les solutions de l’agrivoltaïsme
Grison, C., Cases, L., Le Moigne, M., Hossaert-McKey, M. (2021). Photovoltaïsme, agriculture et écologie. De l’agrivoltaïsme à l’écovoltaïsme.
IDELE (2020). Energies renouvelables : les solutions à la ferme. Etudes, Analyses, Stratégie. Collection – Dossiers techniques de l’élevage
IDELE (2021). L’agrivoltaisme appliqué à l’élevage des ruminants. Guide à destination des éleveurs et des gestionnaires de centrales photovoltaïques au sol
Harchaoui, S., & Chatzimpiros, P. (2018). Can agriculture balance its energy consumption and continue to produce food ? A framework for assessing energy neutrality applied to French Agriculture. Sustainability, 10.
Munoz-Garcia, M.A. & Hernandez-Callejo, H. (2022). Photovoltaics and Electrification in Agriculture. Agronomy, 12, 44
Plateforme Verte (2021). Plateforme pour un agrivoltaisme vertueux. Recommandations.
SER (2020). L’énergie photovoltaïque dans le monde agricole
Solagro (2020). Les parcs solaires photovoltaïques au sol consomment-ils des terres agricoles ? SOLAGRO, pour Enercoop, Energie Partagée et Terre de liens : https://decrypterlenergie.org/les-parcs-solaires-photovoltaiques-au-sol-consomment-ils-des-terres-agricoles
SolarPower Europe (2021). Agrisolar. Best Practices Guidelines. Version 1.0
Terre de liens (2022). Etat des terres agricoles en France. Tout doit disparaïtre ?
Toledo, C. & Scognamiglio, A. (2021). Agrivoltaic Systems Design and Assessment: A Critical Review, and a Descriptive Model towards a Sustainable Landscape Vision (Three-Dimensional Agrivoltaic Patterns). Sustainability, 13
Visit complementary to the interviews
April 2022 – Akuo Energy site at the Broussan sheepfold. Fixed photovoltaic panels with production of apricot, cherry and table grapes.
Interviewees
Nom | Structure |
Adrien ALEXANDRE | Shell |
Xavier BODARD | Davele |
Thibault BUSTOS | VSB Energies |
Alexandre CARTIER | Sun’Agri |
Damien FUMEY | Sun’Agri |
Thibault GRANGE | Agri Terra |
Ronald KNOCHE | Remtec et France AgriVoltaisme |
Lucile NIEF, Lucas OMEZ | Davele |
Jérôme PAVIE | Idele |
Nicolas PISTRE | Urbasolar |
Blandine THUEL | Acte Agri Plus |
Gilles van KEMPEN | FFPA |
Adrien VASSILEVSKY | ADEME |
1 thought on “Agrivoltaism – solar power to conquer agriculture?”